-
Fires pose significant threats to human life and property, while also inflicting substantial damage on ecosystems, resulting in severe disasters[1]. Recently, a fire in the industrial sector of northwest Birmingham, UK, incurred economic damages totaling hundreds of millions of pounds. Presently, managing fire risk has become a major global challenge, with fire safety receiving heightened attention. Each year, forest fires release around 1.5 billion tons of CO2 into the atmosphere, significantly intensifying global warming[2]. With the advancement of modernization, diverse polymeric materials have found widespread applications in various fields such as aerospace, construction, and electronic technologies. Nevertheless, the inherent flammability of polymers poses considerable challenges to fire prevention and control measures[3−5]. Consequently, there is a pressing necessity to establish effective and practical fire safety strategies to reduce the impact of fire-related disasters.
Fire detection and alarm systems are essential and effective strategies for mitigating fire risks. At present, conventional fire-warning devices primarily comprise temperature sensors, smoke detectors, and infrared sensors[6]. Nevertheless, these devices exhibit certain limitations, including prolonged alarm response times (exceeding 100 s), inaccurate signal detection, and degradation or destruction of material properties at elevated temperatures, which prevent the issuance of alerts and hinder effective early warning capabilities[7]. Therefore, there is an urgent need for innovative intelligent fire-warning sensing systems capable of sensitively detecting signals and rapidly identifying fire incidents. Such advancements are essential for facilitating the prompt evacuation of personnel and efficient fire rescue operations.
Increase in temperature is a typical characteristic of fire incidents. Consequently, temperature-sensitive materials are utilized to develop sensors for application in fire-warning systems. Wu et al.[8] developed an intelligent fire-alarm sensing device by applying a graphene oxide (GO)/silicone layered coating to various combustible materials, achieving ultra-fast warning responses (within 3 s). Due to the exceptional thermal reduction properties at elevated temperatures, as well as its unique surface structure, GO is extensively employed in intelligent fire-warning systems. Wei et al.[9] reported the development of a GO/nitrocellulose film that exhibits outstanding flame-retardant properties, rendering it suitable for applications in smart home fire-warning devices, including paints and wallpapers. Furthermore, Xue et al.[10] synthesized a composite utilizing GO and ammonium polyphosphate as a flame retardant for fabric surface coatings. The fabrics treated with this flame-retardant coating demonstrate a high limiting oxygen index (LOI) and stability, indicating their potential suitability for intelligent firefighting uniforms. In addition, various novel fire-warning materials are developed and applied, including carbon nanotubes (CNTs), metal oxides, MXenes, biomass materials, thermochromic materials, shape memory/phase change materials, and certain self-powered materials. Most of these materials exhibit temperature sensitivity, enabling a more responsive fire-warning detection.
In recent years, the rapid advancements in 3S (Remote Sensing, Geographic Information System, Global Positioning System) technology and the Internet of Things (IoT) have resulted in the deployment of various intelligent fire alert systems. For instance, IoT-based real-time forest fire early warning systems[11], and smart home fire detection systems[12] were effectively implemented. In the context of intelligent systems, it is particularly important to achieve multifunctionality to adapt to complex real-world scenarios. Concurrently, attention is given to the large-scale production and application of materials to meet practical demands. This work investigates the warning mechanisms of various fire-warning materials by categorizing them into externally powered fire detection materials based on electrical signal sensing, self-powered type, phase change/deformation, and color change observation. It elaborates on their advantages and drawbacks and introduces their practical applications in the field of fire-warning.
-
Intelligent fire detection sensing materials can sense external environmental changes and convert these states into various signals for effective warning responses. Currently, a crucial method for fire detection is achieved via temperature monitoring[13]. In 2016, Dong et al.[14] first reported the use of GO in fire detection. The GO-HCCP flame-retardant paper shows increased conductivity at high temperatures, and upon exposure to fire, the micro-LED connected through the circuit is lit up and remains functional in the flame. This flame-retardant graphene paper can be utilized in simple alarm devices, establishing a solid foundation for the application of GO in fire detection. Following this, GO-based fire detectors have become a major research focus in firefighting, with several other materials including externally powered fire detection materials based on electrical signal sensing, self-powered fire-warning sensing materials, thermochromic materials, and shape-memory/phase-change materials being reported[15]. Recently, self-powered warning materials such as thermoelectric (TE) and triboelectric nanogenerators (TENGs) have attracted widespread interest[16], as depicted in Fig. 1.
Figure 1.
Research progress of sensing materials for fire warning. The cross-linked network of Tri-molecular compounds containing GO[17]. Structure diagram of C-MXene-coating in the early stage of a fire[18]. Conductive networks are formed by the carbonization of CB@KF[19]. The mechanical flexibility of Ag@Fe3O4-MS[20]. Nylon-cotton (NYCO) blend fabrics[21]. The phase change gel embedded in a polydimethylsiloxane (PDMS) encapsulant[22]. Using LED indicators to evaluate the conductivity of the sample in its initial state, stretched state, and shape-restored state[23]. Schematic illustration of the high-temperature or early fire-warning process of the assembled sensor[22]. Early fire-warning processes of PMS color change sensor and the remote monitoring alarm APP designed by the image recognition algorithm[24]. Design inspiration for molecular sensors[24]. The color of the PMF at 20 and 80 °C[25]. Mechanisms of the three types of heat-to-electricity energy conversion[16]. Emergency Gesture (E-TENG) sensor and Gait recognition (G-TENG) sensor[26].
Externally powered fire-detection materials based on electrical signal sensing
-
Presently, research on fire detection materials primarily concentrates on identifying and responding to temperature changes during a fire, translating these temperature signals into transmittable electrical signals to alert the external environment. Researchers have widely investigated the use of nanomaterials (including GO, MXene, CNTs), metal oxides, and biomass-based materials in fire detection[7,26−29].
GO-based fire-warning sensing materials
-
GO is a representative fire detection material. The oxygen-containing functional groups like hydroxyl, carboxyl, and epoxy groups on its surface disrupt the original conjugated network of graphene, keeping it in an insulating state at room temperature (Fig. 2c). In high-temperature conditions, GO demonstrates highly sensitive thermal reduction properties, causing the removal of surface oxygen-containing functional groups, which lowers its resistance and quickly shifts it from an insulating to a conductive state, forming a fire-warning pathway to enable fire-alarm functions[30]. Zhang et al.[17] developed nacre-mimetic structured tannic acid (TA)-GO/ hexa (N-hydroxymethyl-amino) cyclotriphosphazene (HHACP) ternary hybrid paper through an eco-friendly water evaporation-induced self-assembly method. The synergistic action of multiple flame-retardant mechanisms in both condensed and gas phases impart the TA-GO/HHACP paper with exceptional high-temperature stability and flame retardancy, showing stable and extended continuous fire-alarm duration (over 1,600 s). Due to the carbonization of TA molecules and the radical quenching effect, GO exhibits rapid thermal reduction and changes in electrical resistance. This hybrid paper exhibits a fast trigger response time of 0.6 s and a highly sensitive early warning response to pre-fire abnormal high temperatures, responding in 1 s at 250 °C and 19 s at 200 °C. Furthermore, the multiple interactions between TA/HHACP molecules and GO nanosheets endow the resulting TA-GO/HHACP composite paper with mechanical flexibility, offering broad prospects in areas like construction and textiles (Fig. 2a & b).
Figure 2.
(a) Schematic diagram of the preparation process of the nacre inspired TA-GO/HHACP hybrid network[17]. (b) Fire-alarm duration of graphene oxide based nanocomposite paper[17]. (c) Resistance change of TA-GO/H0.5 paper under flame attack and after extinguishing[17]. (d) Tensile stress-strain curves of pure GO paper and various GO/HCPA composite papers[31]. (e) Photo of flame detection process of GO paper and G1H0.50 paper[31]. (f) Process and conceptual design of PGP composite films prepared by water evaporation induced self-assembly method[32]. (g) Snapshot of LED lamp when PGP composite film burns[32].
The rich oxygen-containing groups on the surface of GO sheets lay a solid foundation for their chemical modification, which in turn can effectively facilitate the thermal reduction process of GO, thus improving its response sensitivity in fire detection. Cao et al.[31] incorporated the water-soluble polyamino molecule HCPA, creating a fireproof coating based on HCPA and GO, which was then applied to rigid polyurethane (PU) foam. The inclusion of HCPA facilitates the thermal reduction of GO, resulting in an ultra-quick fire detection response time of about 0.6 s for the fireproof coating. The composite material exhibits a peak heat release rate (pHRR) reduced by approximately 60% compared to PU foam, with an LOI of 36.5%, indicating its superior flame retardancy. Due to its quick response time and fireproof properties, this composite foam demonstrates promising application prospects in fire detection (Fig. 2d & e). Enhancing the stability of GO through molecular modification and functionalization can confer sustained fire-alarm capabilities to GO-based fire detection materials, which is crucial in practical urban fire emergency applications. Zhang et al.[32] developed an eco-friendly composite film material composed of polyvinyl alcohol (PVA), phytic acid (PA), and GO (PGP composite film) using a straightforward evaporation self-assembly method, as illustrated in Fig. 2f. Under sustained high-temperature heating, the rapid dehydration and graphitization of the PGP composite material induce swift deformation and resistance change, allowing for a sensitive and quick response at relatively low temperatures (150−400 °C), as illustrated in Fig. 2g. Additionally, the hydrogen bonding interactions between polyvinyl alcohol, phytic acid, and GO enhance the thermal stability of PGP, thus providing the composite material with an ultra-long continuous fire-alarm capability of over 2,400 s. Furthermore, the synergy between PA and GO imparts the composite material with outstanding flame retardancy and mechanical strength. The PGP composite material shows essential potential for applications in areas like construction and forest fire prevention.
Nevertheless, GO-based fire detection materials still exhibit several limitations in practical applications. Firstly, the thermally induced resistance change in GO at high temperatures is irreversible, rendering GO-based fire detection materials non-reusable. Secondly, GO's low thermal stability makes it prone to decomposition in high-temperature environments, requiring the introduction of other materials for modification, thereby further increasing practical application costs. Moreover, the high thermal reduction temperature of GO hinders accurate temperature sensing at the early stage of fire.
MXene-based fire-warning sensing materials
-
MXene is a new type of two-dimensional nanomaterial that features a unique layered structure, outstanding catalytic carbonization properties, and strong thermal stability[18,33,34]. Under high-temperature conditions, MXene undergoes thermal oxidation to form TiO2 and generate electron transitions, leading to changes in the material's resistance. This change in conductivity is reversible (Fig. 3a, b). Consequently, MXene-based fire detection materials possess the desirable function of repeated cyclic warnings. Zhang et al.[32] developed a multifunctional coating with a heterogeneous structure by electrostatically assembling silver nanowires (AgNW@BP) with MXene nanosheets and combining them with bio-based sodium alginate. Upon exposure to flame, MXene nanosheets thermally oxidize to form a TiO2 network, effecting a sensitive resistance transition from an insulative to a conductive state and creating a conductive pathway. This imparts the cotton fabric with the capability to achieve ultra-fast fire detection within 2 s. Additionally, this heterogeneous structure coating features a repeatable warning function, indicating broad potential for practical applications in fire warning.
The TiO2 layer produced by the thermal reduction of MXene under high-temperature or flame conditions demonstrates catalytic carbonization properties. The dense char layer formed can effectively impede heat transfer and isolate oxygen, simultaneously reducing the release of flammable gases[35]. Consequently, MXene-based fire-warning materials typically exhibit high flame-retardant properties. Embedding MXene into polymers can markedly improve the flame-retardant effectiveness of the composite materials. Ma et al.[36] developed a sandwich-type fire-alarm wallpaper (FAW) composed of MXene, lignocellulosic nanofibers (LCNF), and ammonium polyphosphate (APP) through a vacuum alternating self-assembly method. This FAW demonstrates ultra-rapid fire response-ability, capable of issuing a fire alarm within 0.32 s of flame exposure (Fig. 3c). The incorporation of APP in FAW promotes the carbonization capability of the LCNF layer and synergizes with MXene's physical barrier and catalytic carbonization effects to achieve thermal insulation and oxygen blocking in both gaseous and condensed phases, thereby slowing down MXene's thermal oxidation reaction and facilitating a sustained alarm function (> 3,000 s). Additionally, the synergistic flame-retardant mechanism between APP and MXene enables FAW to retain high structural integrity during a fire. Furthermore, this wallpaper shows exceptional mechanical flexibility and eco-friendly properties, thus holding significant potential for application in residential decoration.
In practical use, MXene still presents some limitations. Firstly, MXene is susceptible to oxidation in air, potentially impacting its fire-warning response performance. Moreover, MXene often requires combination with other materials for modification or pretreatment in applications like warning sensors, resulting in a complex and costly preparation process, which restricts its potential for large-scale application. In addition, the scalability of high-quality MXene fabrication technologies remains insufficiently mature, and most methods can only achieve fire-warning capabilities within limited areas. Moreover, some related synthesis processes involve the use of hazardous reagents, which contradicts the principles of sustainable development. Furthermore, the reproducibility and stability of MXene processing require significant improvement.
CNTs-based fire-warning sensing materials
-
CNTs demonstrate superior electrical conductivity over a broad range of temperatures[37]. Similar to the operational principles of the previously discussed GO-based fire-warning sensing, CNTs exhibit changes in resistance when exposed to high temperatures or flames. Additionally, due to their unique hollow linear crystalline network structure, CNTs possess exceptional thermal and electrical conductivity. These characteristics endow CNTs with a rapid response capability to temperature. However, such excellent conductivity might cause false alarms or insensitivity in fire-warning applications. Hence, appropriate pretreatment to moderately increase their resistance is essential for developing sensitive and reliable CNTs-based fire-warning sensors. Amino group modification is a widely used and effective pretreatment method[38]. Wang et al.[39] utilized amino-functionalized carbon nanotubes (A-CNT), polyethylene glycol-modified wood (P@MW) flour composite phase change material, and calcium alginate (CA) to prepare a fire-warning aerogel (P@MW-A-CA) through the freeze-drying method. When exposed to flames, the resistance of P@MW-Ax-CA exhibits a sharp decrease due to the rapid removal of amino groups from the A-CNT surface, which results in the formation of a continuous conductive pathway within the aerogel. This aerogel achieved a quick fire-warning response of 2.03 s at a low temperature (150 °C). The surface dehydration and carbonization of P@MW-A-CA confer excellent flame-retardant properties. By encapsulating phase change material polyethylene glycol (PEG) within the aerogel, the material can absorb heat through reversible phase transitions at high temperatures, demonstrating excellent temperature buffering capabilities, thereby holding great potential for applications in fire safety protection (Fig. 4a). The introduction of polymers can also achieve effective fire warning[40]. Xia et al.[19] combined carbon black nanoparticle cotton fiber with CNTs to develop an intelligent fire-warning cotton fabric coating, as illustrated in Fig. 4b. This coating can form a dense char layer and continuous conductive network when exposed to high temperatures or flames, enabling a rapid-fire response (around 4 s, Fig. 4c). The formation of a dense char layer on the surface contributes to the excellent fire-resistant properties of the coating. Under high temperatures or fire conditions, the shell of multifunctional carbon black nanoparticles (CB@KF) decomposes to produce phosphoric acid, which facilitates the carbonization of CB@KF and promotes the formation of additional aromatic carbon. This process is beneficial for establishing a continuous conductive network, thereby enabling the coating to achieve a rapid and sensitive fire-alarm response. Compared to conventional fabrics, the composite fabric's LOI increases from 18% to 25%.
Figure 4.
(a) PEG-@ wood powder/carbon nanotubes/calcium alginate composite aerogel and its application in firefighting clothing[39]. (b) CB@KF-CF fire-alarm mechanism and flame retardant mechanism scheme[19]. (c) XPS survey spectra of the residual chars of CF and CB@KF-CF after fire-warning test[19].
Fire-warning materials based on CNTs exhibit ultra-sensitive warning capabilities following surface modifications. Consequently, they become well-suited for applications in fire-alarm sensors. Due to the high conductivity of CNTs, they cannot be directly utilized in fire-alarm sensors. Instead, pre-surface modification and oxidation treatments are necessary for the carbon nanotubes. This requirement complicates the processing of CNTs, increases production costs, and impedes their practical application in large-scale manufacturing. Furthermore, despite the potential advantages of CNTs in polymer composites, their dispersibility and interfacial adhesion are often suboptimal. Consequently, enhancing flame-retardant performance frequently compromises the mechanical properties of the polymer composites.
Metal-oxide based fire-warning sensing materials
-
Semiconductor materials are extensively applied in the sensor field owing to their excellent sensitivity, stability, and reproducibility. The number of charge carriers within a semiconductor varies at elevated temperatures, resulting in a transition in its electrical resistance. This reversible thermal conductivity characteristic of semiconductor materials enables their application in fire-warning sensors. Recently, the research and development of novel semiconductor materials have been aimed at improving their thermosensitive properties to achieve more precise temperature detection and fire monitoring[41,42]. During this process, metal oxides have found extensive applications in fire-warning systems. Some transition metal oxides possess catalytic carbonization characteristics, which allow them to form dense char layers under flame exposure, effectively blocking heat transfer, suppressing the release of pyrolysis products, and thereby endowing the materials with excellent flame-retardant properties. Zhang et al.[43] developed a 'sandwich' structured fire-warning coating composed of PEI/APP/Ti3O5 using the layer-by-layer self-assembly technique, as indicated in Fig. 5a. Due to the temperature-induced phase transition behavior of Ti3O5, this coating is insulating at room temperature but exhibits a resistive response with increased conductivity under high temperatures, achieving a relatively low thermal response temperature (190 °C). Under exposure to an alcohol lamp flame, this coating exhibits a sensitive alarm time (around 3.78 s) and a prolonged operational duration (16 min). Additionally, Ti3O5 catalyzes the formation of a char layer at elevated temperatures, imparting excellent flame retardancy to the coating. Furthermore, given the reversibility of this metal-insulator phase transition behavior, the coating can achieve outstanding cyclic warning functionality, demonstrating wide adaptability for fire-warning applications on substrates such as wood and fabrics (Fig. 5b). Metal oxides are generally deposited onto substrate surfaces to form coatings for fire-warning applications. However, the low mechanical flexibility of metal oxides usually makes it difficult for them to be used independently as coatings. As a result, they are often combined with adhesives (such as resins and colloids) to improve the adhesion and bonding strength between the coating and the substrate. Zhang et al.[20] developed a thermosensitive fire-alarm fabric based on silver flakes/Fe3O4 nanowires using radiation graft polymerization and sol-gel methods. At room temperature, this fabric remains insulating, but it shows high conductivity after heat treatment. It can achieve rapid (less than 2 s) and sustained fire-warning functionality for over 15 min at a relatively low response temperature (100 °C), with the capability for repeated use. Furthermore, Ag@Fe3O4-MS displays excellent mechanical flexibility and stability (Fig. 5c), offering new perspectives for the development of wearable intelligent fireproof textiles.
Metal oxide-based fire-warning materials possess outstanding thermal stability, demonstrate sensitive fire detection responses, and can deliver prolonged continuous alarms. However, the resistance of most metal oxide semiconductors exhibit a non-linear relationship with temperature, which complicates precise temperature detection. Furthermore, the high costs and intricate manufacturing processes of certain metal oxide materials may pose limitations to their practical applications.
Biomass-based fire-warning sensing materials
-
Within the context of green and sustainable development, biomass-based materials have attracted considerable attention owing to their low cost, ease of processing, and eco-friendly characteristics, especially in fire-warning applications[33,44]. Zheng et al.[45] developed a biomass-based carbon nanofiber (CNF) by incorporating lignin molecules, as illustrated in Fig. 6a. At low temperatures, CNFs act as insulating materials, but in the presence of flame or high temperatures, they can rapidly undergo thermal reduction, transitioning to a highly conductive state (Fig. 6b). Regarding fire-warning, CNF-based sensors demonstrate a low thermal response temperature (< 100 °C), rapid response capability (2 s), and can provide continuous and stable alarm signals (> 5 min). Furthermore, CNFs exhibit high flame retardancy and stability (Fig. 6c & d). The incorporation of ionic compounds or crosslinking agents into biomass-based materials can impart excellent thermally-induced resistive changes and flame retardancy to the composites, enhancing their fire-warning sensing characteristics. Liu et al.[46] developed a silk fibroin (SF)/Ca2+ fire-warning ionic skin utilizing biomass (SF) and CaCl2. With increasing temperature, the migration rate of water and ions in the ionic skin continuously increases, resulting in a rapid decrease in resistance, exhibiting excellent thermally-induced resistive response characteristics. Additionally, under high-temperature conditions, the chlorine elements in the ionic skin can quench the chain reactions of HO radicals, further inhibiting the spread of fire. Furthermore, this ionic skin demonstrates excellent biocompatibility and green degradability, aligning with sustainable development goals and exhibiting great potential.
Although considerable advancements have been made in the field of biomass fire-warning sensing materials, several challenges persist. Most natural biomass materials can not be directly applied due to their inherent flammability; thus, they are typically subjected to chemical modifications to achieve the desired performance characteristics. This process complicates the manufacturing procedures of fire-warning sensors. Furthermore, the extraction techniques for biomass derivatives require further development and optimization. To achieve integrated fire protection that encompasses early warning and flame retardancy in the later stages, significant technical challenges remain. Additionally, the stability of bio-based materials in practical application scenarios needs enhancement, as this can lead to the unreliability of fire-warning signals. In a nutshell, current research technologies pertaining to bio-based materials suitable for fire-warning applications urgently require further enhancement and improvement.
Self-powered fire-warning sensing materials
-
Externally powered fire-warning sensing materials typically require connection to an external power source in applications. Such devices, which rely on external power sources, may be affected by poor battery contact or power interruptions in practical applications. Additionally, harsh outdoor environments may damage external circuits, affecting conductive pathways and compromising the system's fire response performance. This increases the system's complexity and uncertainty. Therefore, researchers are dedicated to developing self-powered fire-warning materials.
TE materials
-
TE materials possess the capability to efficiently convert thermal energy into electrical energy. Based on the Seebeck effect[47], when a temperature gradient exists within the system, carriers migrate from the high-temperature region to the low-temperature region, thereby generating a TE voltage. Furthermore, the voltage produced by TE materials exhibits a good linear relationship with temperature increase, ensuring high accuracy in temperature detection[29]. Moreover, leveraging the reversible characteristics of TE materials, fire-warning systems based on these materials can achieve repeatable responses, thereby providing sensitive and efficient fire-warning capabilities.
Under a temperature gradient, the anions and cations in ionic liquids undergo thermal diffusion. Ionic liquids with high Seebeck coefficients also possess high output voltages, making them superior TE sensing materials for warning applications. Wu et al.[48] used the opposite TE behaviors of two different types of ionic liquids (Fig. 7a & b), 1-ethyl-3-methylimidazolium acetate ([EMIm][Ac], p-type) and 1-ethyl-3-methylimidazolium bis (trifluoromethylsulfonyl) imide ([EMIm][TFSI], n-type) to develop a self-powered green chip for forest fire-warning, as illustrated in Fig. 7c. This TE chip can generate high output voltage even at low temperature differences, thereby producing sensitive early warning signals for fires and demonstrating promising potential in the field of wireless fire detection.
Figure 7.
Schematic illustration of ion desorption of (a) [EMIm][TFSI], and (b) [EMIm][Ac][48]. (c) Typical fabrication process of the single TE unit, TE module based on the connection of [EMIm][Ac] (p-type) and [EMIm][TFSI] (n-type) TE units in series[48]. (d) Design strategy of MXene/CCS@CF; schematic illustration of the application for MXene/CCS@CF including temperature sensing, fire-warning, flame retardancy, piezoresistive sensing, and joule heating performance[50]. (e) The etching process of MXene[50]. (f) Preparation mechanism diagram of OSA[50]. (g) Schematic view of the SS/OSA@MXene TE fiber-based self-powered high-temperature warning sensor simultaneously realized the early high-temperature warning and self-healing functions without external power supply in firefighting clothing[50]. (h) Synthesis rout of MXene and UPC. Fabrication route of MFNC[50].
MXene materials have also garnered significant attention for their exceptional electrical conductivity and TE properties[49]. Owing to the reversible TE properties of MXene, MXene-based functional fibers can be reused, demonstrating significant potential in the field of smart wearable devices. Jiang et al.[50] developed a self-healing TE fiber (silk sericin (SS)/oxidized sodium alginate (OSA) @MXene) using a coaxial wet spinning method, which is suitable for smart firefighting suits (Fig. 7e & f). Firefighting suits integrated with this TE fiber can achieve sensitive fire responses across a broad temperature range of 200−400 °C. Under flame conditions, this material can generate a TE voltage and issue a fire alarm within 1.17 s. Additionally, the firefighting suit is reusable, making it suitable for continuous fire-warning (Fig. 7g). The dynamic covalent bonds between OSA and SS endow the TE fiber with excellent mechanical properties and self-healing capabilities (Fig. 7h). This property significantly enhances the durability of the firefighting suit, advancing the field of fire safety (Fig. 7d). Wang et al.[51] utilized an eco-friendly self-assembly technique to combine MXene nanosheets with carboxymethyl chitosan (CCS), successfully creating a fireproof cotton fabric, MXene/CCS@CF, which can achieve rapid fire responses under high-temperature or flame conditions (less than 10 s). Additionally, the synergistic carbonization between MXene and CCS provides the fabric with outstanding flame retardancy, with an LOI as high as 45.5% in vertical burning tests. Owing to the excellent TE properties of MXene, this multifunctional fabric shows an approximately positive linear relationship between output voltage and temperature during heating, allowing for precise temperature detection. Moreover, the adjustable TE properties of MXene/CCS@CF make it well-suited for the development and enhancement of portable heaters within the rapidly advancing IoT landscape.
Inspired by the structure of skin, Zeng et al.[52] developed a multifunctional flame-retardant nanocoating (MFNC) with sensitive piezoresistive sensing characteristics. This intelligent coating integrates the flame-retardant effects of montmorillonite (MMT) and MXene, showcasing excellent flame-retardant properties with a LOI as high as 40.0%, and can achieve rapid fire response within 4 s. Most coatings degrade over time due to environmental factors or external forces, inevitably leading to diminished flame-retardant performance. However, this flame-retardant coating leverages multiple hydrogen bond interactions between 2-ureido-4[1H]-pyrimidinone cellulose (UPC) and MXene's excellent conductivity, endowing it with self-healing capabilities that allow it to restore its original performance within 24 h after damage. The self-healing innovation of MFNC offers extensive application prospects in firefighting and smart manufacturing. MXene-based functional fibers can also be incorporated into firefighting suits[53].
TE materials can achieve sensitive fire-warning responses without external power sources and can be reused, making them ideal fire-warning sensing materials. However, current fire-warning systems based on TE materials still face challenges such as high raw material costs, complex structural designs, and unstable fire responses, necessitating further research and improvements[16].
TEC materials
-
The operational principle of thermal batteries is based on the temperature dependence of electrochemical oxidation-reduction potentials, which enables efficient thermal-to-electrical conversion. This unique characteristic holds significant promise for applications in fire-alarm systems. In their study, Yu et al.[54] developed a concentration gradient thermal battery, known as a thermocell (TCC), utilizing a K3Fe(CN)6/K4Fe(CN)6 aqueous electrolyte. The introduction of a concentration gradient significantly enhances the open-circuit voltage (Voc) of individual cells, increasing it from an initial value of less than 140 mV to over 500 mV, thereby achieving a substantial output Voc.
To address the issue of concentration gradient reduction resulting from battery discharge and spontaneous diffusion, the authors incorporated a heat-triggered membrane (HTM) based on hydrophobic phase change materials. This membrane acted as a 'switch', regulating the ionic concentration gradient and mitigating problems associated with an unstable power supply. The resulting heat-triggered TCC remains in a dormant state under normal conditions. When exposed to high temperatures or flames, the HTM undergoes a phase transition, activating the 'switch' and generating a high Voc, which effectively triggers fire-alarm responses.
Additionally, the authors integrated a forest fire early warning system with wireless sensors and Bluetooth technology, leveraging the heat generated by the fire itself to facilitate timely alert responses. When a fire occurrs, alarm signals can be rapidly transmitted to mobile devices. This automated and remote self-powered warning system provides a promising approach to achieving early fire detection and prevention.
TENG-based fire-warning materials
-
The operating principle of TENGs is predicated on the coupling effect of contact electrification and electrostatic induction. When two dissimilar materials come into contact, charges are generated on their surfaces[55]. According to the triboelectric effect, these charges transfer between different polarities, forming a potential difference. When a TENG is connected to an external circuit, electrons flow between the two electrodes to achieve potential equilibrium, thereby generating an output electrical signal[56]. Consequently, TENGs can harvest mechanical energy from the external environment and convert it into sustainable electrical energy. In the external environment of a fire incident, various forms of mechanical energy exist, such as wind energy, object movement, and human activity. Therefore, TENGs show tremendous potential in fire-warning applications.
Leveraging their exceptional energy harvesting capability, TENGs can utilize the mechanical energy generated during human movement for fire sensing and warning, exhibiting significant application potential in portable and wearable electronic devices. He et al.[57] employed traditional wet spinning technology to combine Fe3O4 nanoparticles (Fe3O4NPs), AgNWs, and CA, successfully fabricating a thermally induced ultra-light conductive aerogel fiber, as illustrated in Fig. 8a. Utilizing TENG as a power source, they developed a self-powered fire- alarm electronic textile (SFA e-textile) based on this aerogel fiber (Fig. 8b). This textile can be integrated into firefighting suits to achieve temperature sensing across a wide range of 100 to 400 °C and can issue timely warning signals before a fire occurs. Furthermore, the textile exhibits repeatable fire-alarm capabilities. TENG-based self-powered fire-warning sensors can be used to monitor firefighters' movements at fire scenes, facilitating motion monitoring and early warning, thereby enhancing their safety. Sun et al.[26] designed two types of TENG sensors capable of monitoring firefighters' movements through the simple assembly of patterned MXene ink electrodes (MiEs) and fire-resistant high-temperature triboelectric materials, as depicted in Fig. 8c. Among them, the E-TENG can identify basic gestures by detecting the bending state of the arm, while the G-TENG can sense and identify various gaits, both with high recognition accuracy. These TENG sensors possess the capability to monitor motion states and exhibit considerable potential for enhancing emergency evacuation and rescue operations during high-temperature fire incidents.
Figure 8.
(a) TIC-AF and SFA electronic textile manufacturing schematic[57]. (b) Photographs of TIC-AF and TIC-AF surfaces and cross sections manufactured with different diameters[57]. (c) The construction drawing of TENG sensors for monitoring human motion on fireground. Patterned inks electrodes: MXenes-inks; the E-TENG sensor and G-TENG sensor[26]. (d) Schematic diagram of MC-TENG for collecting kinetic energy of branch shaking[58]. (e) Application scenarios and overview of TD-TENG for wind energy harvesting[59]. (f) Schematic diagram of double-layer TD-TENG based on six TENG units[59].
Furthermore, TENGs can directly capture various forms of energy from the natural environment, such as vibrations, wind, and air convection, and convert these energies into electricity to facilitate fire-alarm responses. Pang et al.[58] reported a novel multilayer cylindrical TENG (MC-TENG) that collects kinetic energy generated by wind-induced branch movements to power fire response systems (Fig. 8d,e). Gao et al.[59] developed a turbine disk-type TENG (TD-TENG) designed to capture wind energy, enabling sensitive fire- warnings (Fig. 8e). This turbine disk structure effectively reduces wear between materials during high-speed rotation, significantly improving the durability of forest fire-warning systems and extending the practical application potential of TENG-based distributed energy harvesters, as presented in Fig. 8f.
Currently, the primary challenge faced by TENG-based fire-warning devices is the wear of materials due to contact friction, resulting in insufficient mechanical durability of fire-warning systems. Moreover, most TENGs struggle to operate effectively at high temperatures. Therefore, future research should focus on developing fireproof coatings suitable for TENGs.
Phase change/deformation fire-warning sensing materials
-
Fire incidents are usually accompanied by significant temperature changes or exothermic phenomena. Phase change materials (PCMs) change their mechanical state in response to temperature variations, making them widely applicable in fire-warning systems. For example, highly crystalline polymers transition to a liquid state when heated above their melting point. These solid-liquid phase change materials are suitable for fire-warning systems due to their excellent stiffness variation properties. Currently, phase change materials are typically integrated with alarm lights and power sources to form fire-warning sensing systems[60].
PEG is a commonly used phase change material. Zhao et al.[22] prepared a high-temperature warning phase change gel by forming a polyacrylic acid (PAA) cross-linked network within the phase change PEG melt via in situ polymerization (Fig. 9a). Owing to PEG's exceptional thermal responsiveness and its reversible phase transition from crystalline to molten state at high temperatures (and vice versa at low temperatures), the gel achieves thermally sensitive resistance changes, effectively enabling fire warning (Fig. 9b−d, g). Meanwhile, the cross-linked PAA network serves as a supporting framework, preventing PEG leakage (Fig. 9e). Therefore, even under high temperatures exceeding PEG's melting point, the phase change gel retains its flexibility while maintaining its original structure (Fig. 9f). Additionally, PCMs possess the ability to actively store heat through latent heat and release this energy upon reaching a specific critical phase transition temperature. These materials have become important components for energy storage and release due to their efficient heat absorption and release during phase transitions, particularly showing exceptional application prospects in the fields of energy saving and environmental protection.
Figure 9.
(a) Preparation of phase change gels and their mechanical properties in soft and rigid states[22]. (b) Macroscopic and (c) microscopic images of the crystallization and re-melting process of the phase change gel[22]. (d) Digital images showing the stiffness difference for the phase change gel between the rigid and soft states[22]. (e) A comparison of heat conduction under two different states at 90 °C[22]. (f) Demonstration of the shape memory effect of the phase change gel[22]. (g) Schematic of the highly tunable and reversible features of the phase change gel[22]. (h) Electrical resistance change of the integrated circuit as a function of temperature[22].
Shape memory polymers (SMPs) have been utilized in fire-warning systems. When exposed to flames or high temperatures, SMPs can rapidly alter their shape. Similar to phase change material-based fire alarms, SMP fire-warning devices are usually composed of two parts: shape memory polymers and conductors. Under the influence of flames, the polymer undergoes stretching, contraction, and bending, causing the conductor to shift position, ultimately forming a closed circuit to trigger the fire alarm[61]. Chen et al.[62] reported a copolymer containing PEPN, a monomer that incorporates both aniline and phenylacetylene. Above the transition temperature, the copolymer undergoes shape changes. When the temperature drops below the transition temperature, the movement of the copolyester segments is frozen, enabling the polymer to maintain a fixed temporary shape. A fire-alarm circuit can be constructed based on this shape-changing copolymer. The copolymer's excellent shape memory properties and self-healing ability under thermal stimulation enable it to achieve rapid and sensitive fire-warning functions, with response times of less than 5 s. Jia et al.[23] developed an intelligent polymer conductor capable of switching between non-conductive and conductive states (OFF-to-ON) using thermosensitive SMPs via chemical deposition techniques. As the temperature changes, the shape of the SMP changes accordingly, leading to changes in its conductivity, thereby triggering fire-warning signals. This fire-warning device can efficiently provide warnings across a wide temperature range. Its manufacturing process and equipment design are relatively simple, and it utilizes biodegradable materials, aligning with the needs of sustainable development and offering high practical application value.
Besides electrical signal sensing materials, shape-changing materials based on MXene are also applicable to the fabrication of fire-warning sensors. Zhang et al.[63] developed a novel flame-retardant SMPU/MXene deformation paper that can be designed into various shapes and revert to its initial state upon heating, thus forming conductive pathways to issue fire alarms. Additionally, due to the physical barrier properties of MXene, this SMPU/MXene paper can effectively prevent self-extinguishing drips. By adjusting the cross-linked network's structure, the deformation temperature can be precisely controlled, thereby setting a broad response temperature range suitable for different applications to achieve more accurate fire warnings.
Phase change/shape-changing materials are reversible and can be reprocessed, providing convenience in practical applications. Furthermore, by adjusting the polymer network, the critical phase change/deformation temperature can be precisely tuned, thereby achieving reliable and accurate fire warnings.
Color change-based fire-warning sensing materials
-
With the rapid advancement of IoT digital technology and the ongoing updates of electronic devices, digital imaging technology is also evolving. Therefore, the real-time monitoring capabilities of digital cameras, combined with intelligent image recognition technology, can be utilized for video surveillance to achieve remote, visual, and highly accurate fire warnings. Currently, real-time fire monitoring primarily relies on intelligent surveillance and recognition systems that achieve immediate alarm responses by detecting fire or smoke pixels, outperforming traditional smoke/infrared sensors[25]. Thermochromic materials undergo internal molecular structural changes with temperature variations, leading to color changes. Intelligent image recognition systems based on these materials can obtain intuitive early fire-warning signals when the temperature rises (early stages of fire), enabling rapid-fire warnings that facilitate the timely handling of fire hazards.
Inspired by the chemical structure of chlorophyll, Fu et al.[24] designed and synthesized a phthalocyanine precursor molecular sensor (PMS), as illustrated in Fig. 10a. Due to the excellent color-changing properties and thermal stability of phthalocyanine molecules, early fire warning devices based on this sensor can achieve color changes under high-temperature conditions. By integrating real-time video surveillance systems with intelligent image recognition technology, such fire-warning devices can provide reliable and rapid high-temperature fire alerts, as depicted in Fig. 10b. The PMS system provides an effective solution for early fire warning and detection, with its application scope extending to various industrial sectors such as chemical and electrical industries, showing broad application prospects. By combining polyhedral oligomeric silsesquioxane (POSS) with coordination ions, the preparation of thermochromic materials can be achieved. Xu et al.[25] prepared a POSS-metal film using octa (aminopropyl) POSS and metal salt [Cr(NO3)3·9H2O] through solvent evaporation methods. Based on ligand field theory[64], this film undergoes rapid and reversible color changes under high temperatures or flames, thus achieving repeatable fire-warning functions.
Although sensors based on color-changing molecules can quickly detect early fires with the help of intelligent image-monitoring technology, they still exhibit relatively low response temperatures. Moreover, the thermochromic molecules currently applicable to fire warnings exhibit poor flame retardancy. To address these two issues, combining thermochromic molecules with GO can more effectively realize fire warnings. He et al.[28] reported an asymmetric nanocomposite paper made by combining poly (pentacosadiynoic acid)[poly (PCDA)], hydroxyapatite (HAP), and 3-aminopropyltriethoxysilane (APTES)-modified GO. The top layer of poly (PCDA)/HAp allows for thermally induced reversible color changes. APTES molecules effectively enhance the flame retardancy of GO, while the HAp nanowire framework exhibits excellent fire retardancy, ensuring the structural integrity of the asymmetric nanocomposite paper under flames. This nanocomposite paper can achieve rapid fire response (approximately 1 s) and sustained fire warnings at both low (55 °C) and high (159 °C) temperatures.
-
This review summarizes recent advancements in fire-warning sensing materials, categorizing them into four distinct types based on their alarm mechanisms. Over the past decade, researchers have implemented a diverse array of strategies to develop fire-warning devices, which have been widely applied in the field of fire prevention. These innovations include multifunctional fire-alarm papers/membranes, nanocoatings, smart fabrics, and aerogels. Currently, the majority of developed smart fire-warning materials exhibit rapid flame-warning response time (Fig. 11). This advancement facilitates the issuance of timely evacuation signals, thereby enhancing the protection of lives and property. Among them, multifunctional materials based on temperature-triggered resistance transition have attracted increasing attention in recent years, demonstrating promising applications in practical scenarios. These fire-warning materials have achieved several desirable parameters, including rapid flame detection time, low fire-warning temperature, high sensitivity, and stable cyclic warning performance. However, to obtain optimal fire-alarm signals across various application contexts, further development of multifunctional materials that concurrently possess these desired parameters remains essential. Additionally, the aforementioned materials typically necessitate an external power source to generate electrical signals, complicating their practical applications. Most currently reported self-powered fire-warning sensor materials still encounter challenges such as low voltage and low output power. Consequently, there is an urgent need to develop higher-performance self-powered materials to realize high-output power self-sustaining warning systems. Furthermore, phase change and deformation fire-warning materials not only exhibit excellent reusability but also enable precise control of response temperatures through adjustments in phase or shape, making them well-suited for applications in fire safety. Additionally, the thermochromic properties of color-changing materials offer more intuitive fire-warning signals. However, both types of fire-warning sensing materials demonstrate inadequate weather-resistance, which limits their applicability in complex environments and necessitates further optimization to expand their application scenarios. In summary, despite the significant progress made in smart fire-warning sensor materials over the past few years, the aforementioned challenges still need to be addressed. In this section, the possible opportunities will be discussed.
Figure 11.
Comparison of flame detection alarm time. Notes: GO/silicone coating[8]. APP/GO/TFTS coated PU[65]. MPTS-GO paper[66]. GOWR- coated MF sponge[67]. GONR coated MF sponge[67]. GO/BP-MoS2 film[31]. GO/HCPA paper[31]. Silane-f-MXene/PVP coating[33]. PLCNF/gelatin/MXene[49]. PI@MXene aerogel[68]. FGO/CNTs coated WPP[69]. CCS/MMT/A-CNT aerogel[37]. FGO/CNTs coated PU sponge[70]. CB@KF/CNT/PVA coated CF[19]. PEI/APP/Ti3O5 coating[43]. Ag sheets/Fe3O4 NWs coated[20]. TiO2/MMT/CNF coatings[71]. PPI-based carbon nanofibers[45]. SF/graphene skin[72]. Copolymer containing PEPN[62]. CS-NaCl film[73]. Graphene/NC composite[9]. Asymmetric Nanocomposite Paper[28]. OSA and SS TE fiber[50]. MXene/CCS@CF[51]. MMT-MXene coating[52]. FR-TENG[74]. S-TENG[75].
Multifunctional exploration
-
Although significant progress has been made in fire-warning materials and intelligent sensing over the past few years, there are still several challenges that need to be overcome. At present, most fire-warning sensing systems focus on the performance research and application of single materials to achieve flame retardant or warning abilities. However, complex environmental scenarios result in many uncertainties in practical applications, requiring a comprehensive consideration of the various performances of fire-warning systems (Fig. 12).
Figure 12.
Development prospects of fire-warning systems. Recyclability and processability of BRSC Schematic diagram of self-powered[76]. Forest fire- alarm system for spherical standalone TENG (S-TENG) as a wind energy collector[75]. Electrical stability test of the LED arrays under water and oil phase[77]. Wireless conversion diagram of fire signal to Wi-Fi signal for remote monitoring of fire-alarm process[78]. Remote area fire detection system based on Internet of Things[11]. Schematic diagram of multi-point flame cycle detection sensor circuit connection[71].
Multi-mechanism synergistic warning
-
The reliance on a singular warning mechanism presented inherent limitations. The integration of multiple early warning systems complemented one another, thereby significantly enhancing the reliability and stability of the overall system. This approach effectively addressed the shortcomings associated with singular fire-alarm systems, such as higher false alarm rates and lower detection accuracy. By flexibly selecting and combining warning functions, multi-mechanism warning systems can effectively adapt to the requirements of various environments and application scenarios.
Zou et al.[79] developed an intelligent warning cotton fabric through in-situ polymerization of polypyrrole (PPy) combined with MMT and APP. This fabric relies on the characteristics of PPy, featuring dual warning mechanisms of the TE effect, and thermally induced resistance changes. Under the TE effect, when a temperature gradient is applied to the fabric, the voltage and temperature increase show a good linear relationship, enabling the fabric to exhibit accurate temperature sensing capabilities and multiple warning functions at low temperatures (below 100 °C). Meanwhile, the thermally induced resistance change mechanism allows the fabric to quickly issue alarms under high temperature or flame conditions. This composite fabric shows excellent performance in fire prevention, temperature sensing, and fire response, demonstrating broad application potential in the field of fire protection electronics, such as clothing equipped with fire prevention and alarm functions.
In recent years, although GO has been widely applied in the field of fire warning due to its ultra-fast response capability and excellent mechanical properties, the high temperature required for GO thermal reduction (over 400 °C) leads to sluggish reactions, making it incapable of accurately monitoring the heating process before combustion. Therefore, developing GO-based fire-warning devices with low response temperatures (below 100 °C) remains challenging. He et al.[28] combined the advantages of fire warnings based on resistance change and color change to develop a novel dual-mechanism nanocomposite paper composed of poly PCDA, ultra-long HAp nanowires, GO, APTES, and HAp nanowires. The poly PCDA/Hap side can achieve thermally induced responses at low temperatures (30−70 °C), exhibiting reversible color changes from purple to red, thereby triggering a fire alarm. Meanwhile, the GO/APTES/Hap side utilizes GO's thermally induced resistance change mechanism, transitioning from insulative to conductive at high temperatures, with a response temperature of 159 °C. This asymmetric nanocomposite paper can achieve rapid fire-warning responses under both high and low temperature conditions, showing great potential in the practical application of fire-warning systems. Liu et al.[75] proposed a fire-warning system that combines an S-TENG and a polydopamine-modified GO (P-GO) thermosensitive sensor. Benefiting from the impedance matching effect between S-TENG and P-GO, this self-powered system can achieve rapid fire responses in both high and low-temperature environments, providing real-time warnings during the early smoldering and flaming stages of a fire, thereby promoting advancements in forest fire detection technology.
Self-healing capability
-
Current fire-alarm sensors often lack sufficient mechanical adaptability under extreme conditions, making them vulnerable to stretching, bending, and other mechanical deformations in practical applications, leading to breakage. Such damage can disrupt conductive elements or pathways, thereby shortening the system's lifespan, reducing sensing capabilities, and even leading to system failure, severely impacting fire-warning performance. Therefore, endowing fire-warning systems with self-healing capabilities is crucial.
Jiang et al.[50] reported a novel self-healing TE gel fiber constructed from SS and OSA. Through coaxial wet spinning technology, this fiber achieved a fire-retardant self-healing shell layer and a highly sensitive temperature-sensing core layer. Its internal strong and reversible dynamic covalent bonds give it a self-healing rate of up to 89.12%, along with reversible high-temperature warning capabilities. Additionally, the reversible Schiff base reaction between OSA and SS allows the self-healed fiber to maintain excellent tensile strength. This self-healing fiber demonstrates broad application potential in the field of wearable self-powered high-temperature warning sensors. Jia et al.[76] successfully prepared a bio-based polymer by introducing dynamic imine covalent bonds and hydrogen bonds into the cross-linked network of vanillin and tung oil. The presence of multiple hydrogen bonds within the polymer enhances the density of the cross-linked network, thereby imparting excellent mechanical properties to the polymer, enabling it to withstand high tensile strength. Additionally, the high structural compatibility between the covalent bonds and flexible aliphatic hydrocarbon chains within the polymer allows this vitrimer composite material to exhibit excellent self-healing properties. At 120 °C, the bio-based material still maintains high tensile strength after mechanical damage and subsequent repair.
Corrosion resistance
-
In practical applications, factors such as oil stains, rainwater, acidic and alkaline liquids, and organic solvents may corrode the equipment components or conductive pathways of fire-warning systems, thereby impacting their fire-alarm performance. Therefore, improving the system's corrosion resistance is especially important.
Wang et al.[77] developed a fire-warning fabric using ethylene glycol diphosphate, polyaniline, and silver nanoparticles (AgNPs). The composite fabric has a water contact angle of approximately 158° and an oil contact angle of around 153°, exhibiting superhydrophobic and superoleophobic properties. Its surface coating exhibits high electrical conductivity, and due to the synergistic flame retardant effect of silicon, phosphorus, and nitrogen, the coating forms a dense char layer at high temperatures, effectively protecting the conductive network composed of AgNPs and ensuring good stability. This composite fabric demonstrates excellent electrical conductivity under various conditions such as moisture, oil stains, high temperatures, and repeated deformation, showing significant application potential in multifunctional wearable textiles. Kong et al.[80] developed a novel flame-retardant fabric based on aminotrimethylene phosphonic acid (ATMP) and allantoin (CF@AA). They combined PDMS with multi-walled CNTs (MWCNT) and assembled it onto CF@AA, followed by surface sealing treatment, to create a multifunctional smart textile (CF@PM-AA). After surface sealing treatment with PDMS, the smart textile exhibits a superhydrophobic surface and maintains high flame retardancy in both acidic and alkaline environments. Additionally, the TENG based on CF@PM-AA can be effectively applied in wearable fire-warning systems, maintaining its original warning performance under harsh conditions (such as rainy days), significantly enhancing the safety of the warning system.
Integration with intelligent technologies
-
Traditional fire monitoring systems mostly rely on wired connections and manual operations, and they struggle to achieve real-time monitoring in the absence of network connectivity[81,82]. This limitation is particularly prominent in isolated areas, causing significant challenges in fire emergency response. In recent years, with the rapid development and integration of 3S technologies, the use of wireless communication links for data transmission has become more convenient and flexible, making it a smarter and more efficient warning method. Meanwhile, the continuous development of IoT systems have provided a robust network foundation for intercommunication among various devices. This advancement has significantly enhanced the efficiency, performance, and security of smart city construction, driving the development of a series of intelligent fire-warning sensing systems.
Dampage et al.[83] proposed a forest fire detection system based on a wireless sensor network, capable of monitoring multiple parameters such as temperature, relative humidity, light intensity, and carbon monoxide concentration. In the analysis phase, the system employs machine learning regression models to enhance detection accuracy. Once a fire is detected in a specific area, the system sends alert messages to the authorized personnel's mobile phones, enabling them to quickly take fire prevention measures and effectively mitigate the damage caused by the fire. Zhang et al.[84] developed a fire-warning system based on IoT sensors, employing deep belief networks and recurrent long short-term memory neural network methods to predict fires. This system uses an Arduino microcontroller unit to process detection data such as temperature, flame, and smoke, enabling real-time analysis of environmental conditions.
Currently, many smart fire-warning controllers exhibit excellent fire-alarm signal transmission capabilities; however, in practical applications, some fire detectors are prone to false alarms. False alarms not only lead to unnecessary resource consumption but may also cause public confusion. Therefore, analyzing and predicting monitoring data using machine learning algorithms, in conjunction with existing fire models for evaluation, is crucial for enhancing the alarm accuracy of fire-warning systems. Sarwar et al.[85] designed a fuzzy logic-based fire monitoring and warning system. The system uses multiple sensors to make decisions based on four parameters: rate of temperature change, rate of humidity change, flame detection, and time, and it was experimentally validated in a MATLAB environment. This system is capable of achieving high fire-warning accuracy. Additionally, machine learning algorithms enable intelligent warning responses, overcoming the limitations of traditional manual prevention and control while enhancing fire-warning efficiency and accuracy. Based on an IoT architecture, Alqourabah et al.[86] developed an intelligent fire detection system equipped with an automatic sprinkler device, capable of monitoring data such as gas, temperature, and smoke. The system connects to a central processor through a Wi-Fi module and uses the Ubidots platform for data processing. When the monitored parameters reach the set thresholds, the water pump activates, triggering the sprinkler function. Additionally, the system integrates machine learning technology, enabling proactive fire prevention and control, and demonstrates significant practical application potential.
Large-scale practical applications
-
Currently, the research and preparation of fire-warning sensor materials are mainly conducted in laboratory settings, typically testing material performance through small-scale fire scenario simulations. However, real-world applications often involve large-scale forest fires or building fires. Therefore, in large-scale practical applications, many fire-warning materials often struggle to provide effective safety assurance. Thus, it is essential to develop fire-warning systems that can effectively adapt to large-scale applications, facilitating the transition from laboratory research to practical use.
To achieve a fire-warning response, GO must undergo a rapid and complete thermal reduction process, posing challenges for the effective application of GO-based fire-warning coatings on large substrates. Khan et al.[87] prepared a composite flame-retardant coating composed of GO, polydimethyl aminoethyl methacrylate (PDMAEMA), and hexagonal boron nitride (BN), and applied it to cotton fabric. The crosslinking synergistic effect between GO and BN significantly enhanced the coating's durability, allowing it to maintain good warning performance under various conditions such as washing, bending, and abrasion. Additionally, patterning GO, PDMAEMA, and BN with conductive ink allows the distance between any two electrodes to be maintained at the micron level, enabling rapid response in large-scale fire-warning applications. This smart coating demonstrates significant practical application potential in areas such as transportation, housing, and appliances. MXene has garnered widespread attention in the field of fire warning due to its rapid fire response capability and repeatable warning performance. However, both MXene and GO surfaces exhibit a deep black color, affecting their appearance and limiting their application in the decoration industry. Additionally, MXene processing typically requires expensive nanomaterials and complex preparation processes, resulting in high costs for practical applications, which is unfavorable for large-scale production. Shen et al.[71] employed a low-cost and environmentally friendly method to prepare a water-based three-dimensional titanium oxide/two-dimensional montmorillonite/one-dimensional cellulose nanofiber (TiO2/MMT/CNF) layered composite material. The MMT filler in the nanomaterial significantly enhances the flame-retardant performance of the coating, enabling it to maintain structural integrity for an extended period under flame exposure. Benefiting from a good thermally induced resistance change effect, the composite material can achieve rapid fire-warning response (less than 3.5 s) under high temperature or flame exposure. Additionally, adding pigments to the material allows for easy adjustment of the coating's color. This low-cost, color-adjustable nanocoating shows significant application prospects in the field of fireproof decoration and has the potential for large-scale practical applications.
-
This article presents a thorough overview of the advancements in fire-warning sensing materials over the last ten years and classifies them into four categories according to their warning mechanisms: (1) Fire-warning materials that rely on electrical signal sensing without self-powering; (2) Phase change/deformation; (3) Color change observation; (4) Self-powered type. The first category enables quick and sensitive fire-warning responses, but it requires an external power supply. The other types generally have reusable features; however, they continue to confront challenges like high material costs and complicated processing methods. Furthermore, fire-warning systems must be equipped with multiple functionalities, including multi-mechanism synergistic warning, self-healing capabilities, and corrosion resistance, to effectively cater for the practical application demands in complex environments; The integration of intelligent technology with fire-warning materials will enable the developed smart warning system to provide more efficient and secure fire-warning capabilities. In light of actual requirements, it is crucial to create fire-warning systems capable of effectively accommodating large-scale applications to achieve the transformation from laboratory studies to real-world applications.
This research was supported by the National Natural Science Foundation of China (52474245), the Hubei Provincial Natural Science Foundation of China (2023AFD029) and the National Innovation and Entrepreneurship Training Program for College Students (202410497099 and 202410497095).
-
The authors confirm contribution to the review as follows: study conception and design: Ma X, Fang Y; data collection: Wu R, Yang L, Wang Y; analysis and interpretation of results: Yuan B; draft manuscript preparation: Ma X, Fang Y, Wu R, Yang L, Wang Y, Yuan B. All authors reviewed the results and approved the final version of the manuscript.
-
The datasets analyzed during the current study are available from the corresponding author on reasonable request.
-
The authors declare that they have no conflict of interest.
- Copyright: © 2025 by the author(s). Published by Maximum Academic Press on behalf of Nanjing Tech University. This article is an open access article distributed under Creative Commons Attribution License (CC BY 4.0), visit https://creativecommons.org/licenses/by/4.0/.
-
About this article
Cite this article
Ma X, Fang Y, Wu R, Yang L, Wang Y, et al. 2025. Fire warning sensing materials: advances, prospects, and challenges. Emergency Management Science and Technology 5: e005 doi: 10.48130/emst-0025-0003
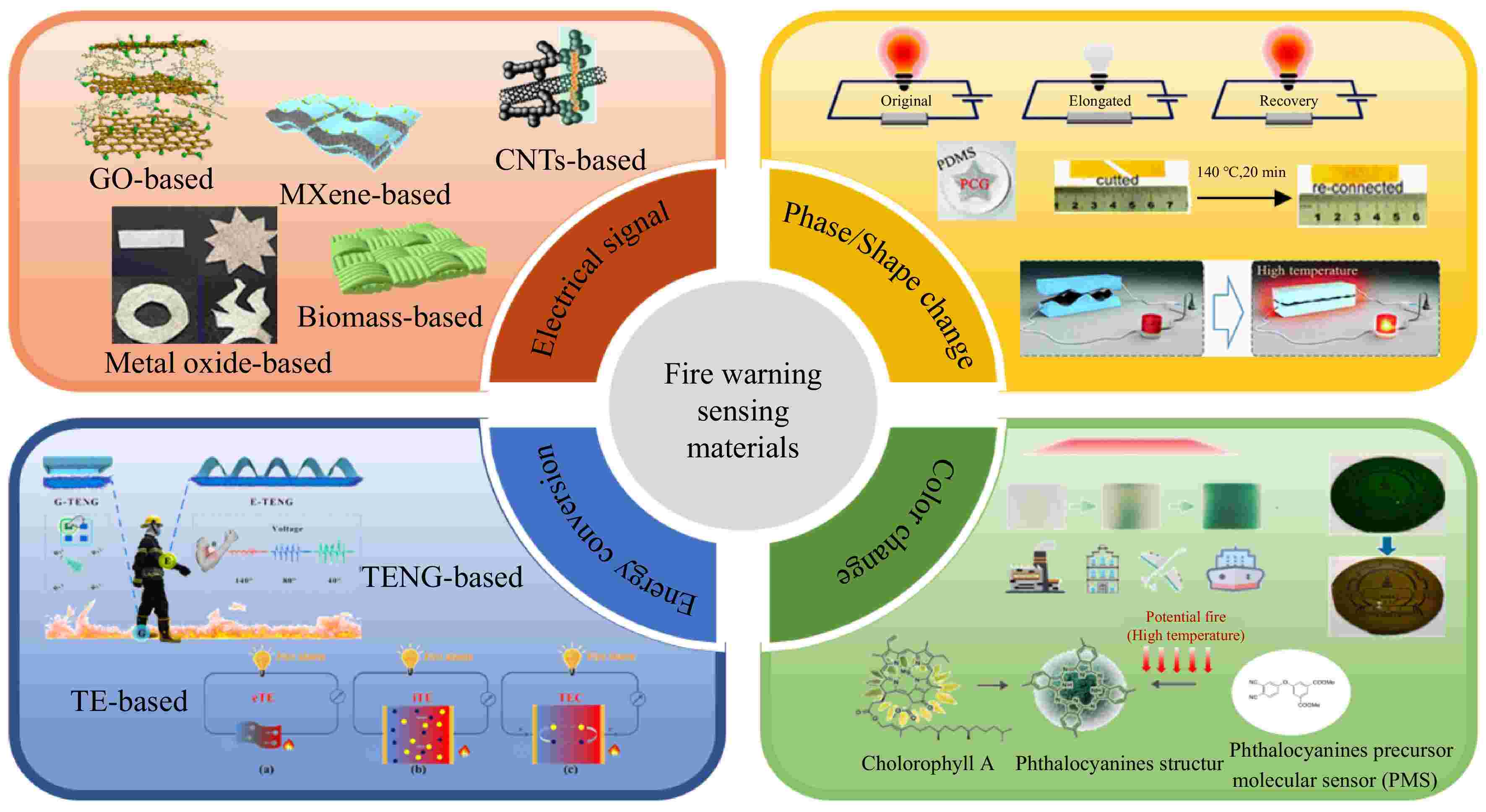