-
Plant response to saline-alkali stress is a significant area of research in plant science. Saline-alkali stress typically results in negative effects due to high salt concentration and high pH in the soil, affecting plant growth and development[1,2]. Soil salinization, as a pervasive environmental stress, poses a serious challenge to global agricultural production, especially in arid and semi-arid areas[3,4]. Saline-alkali stress affects the water uptake capacity of plants, causing osmotic stress. At the same time, high concentrations of ions such as Na+ and Cl- can cause ion toxicity, affecting photosynthesis and respiration in plants[5,6]. In addition, high pH reduces plant uptake of nutrients such as iron (Fe), leading to symptoms like iron-deficiency leaf yellowing[2]. Plants have established mechanisms to cope with saline-alkaline stress by modulating physiological processes, including leaf microstructure, stomatal regulation, photosynthesis, and ion and hormone balance[7,8].
In recent years, research on the enhancement of plant saline-alkali tolerance by Arbuscular Mycorrhizal Fungi (AMF) has made remarkable progress and is constantly being deepened[9]. AMF is widely distributed in saline-alkali soils and can establish symbiosis with over 80% of terrestrial plants, which is considered a biotechnology that can enhance plants' capacity to tolerate saline-alkali[10]. AMF significantly enhances the adaptive capacity of plants in saline-alkali soils by forming a symbiosis with plant roots[11]. This enhancement is achieved by promoting the uptake of nutrients and water, improving the physicochemical properties of inter-root soil, enhancing plant photosynthesis, and inducing plant gene expression[12]. Studies have shown that AMF can promote plant growth and development by enhancing nutrient uptake, improving growth, and regulating endogenous hormones[13,14]. For example, the influence of AMF on photosynthesis and hormone homeostasis in arid environments has been shown to bolster drought tolerance[15]. Additionally, inoculation with AMF has been reported to improve drought tolerance in trifoliate orange by modulating levels of abscisic acid (ABA), gibberellin (GA3), and brassinosteroid (BR)[16]. AMF's capacity to enhance endogenous hormone regulation is well-documented[13,17]. Furthermore, AMF has been shown to mitigate the uptake of sodium (Na+) while enhancing the uptake of essential nutrients like nitrogen (N), magnesium (Mg), zinc (Zn), and copper (Cu), thereby improving saline-alkali tolerance in plants such as Acacia nilotica[18]. Currently, it has been reported that AMF can form a symbiotic relationship with the apple root system to enhance the apple's adaptability to environmental stresses[19]. For example, it was found that inoculation with AMF could enhance the resistance of apple to rusty fruit diseases and replanting diseases[20]. However, most current research on the symbiosis between apples and AMF has predominantly focused on the underground parts and the enhancement of apple resistance to biotic stresses, while relatively few studies have been conducted on abiotic stresses affecting the aboveground parts.
In recent years, domestic and foreign studies on plant salt or alkali damage have mainly focused on single salt or single alkali. In studies of mixed saline-alkali stress, the majority of attention has been given to herbaceous plants, crops, and pasture grasses[21]. At present, scholars have carried out a lot of research on the response of apple plants to saline-alkaline stress, and have made initial achievements. Dwarf and dense planting is the main direction of apple cultivation worldwide. M9-T337 is a superior apple dwarfing rootstock free of viruses, selected by the Netherlands Inspection Service for Horticulture, which is 20% more dwarfing than the traditional M9 rootstock[22]. The rootstock is widely used worldwide, especially in the Northwest Loess Plateau area. Due to its adaptability, distinct trunk, ease of flowering, and high productivity, it has been widely cultivated and applied[23]. However, the combined effects of natural factors such as climate, hydrology and topography, anthropogenic destructive factors such as over-fertilization and irrational irrigation, and the fragile ecological environment in this region have resulted in the gradual accumulation of soluble salts (NaCl and Na2SO4) and alkalis (Na2CO3 and NaHCO3) in the soil, leading to deterioration in the quality of the soil to form saline-alkaline soils, which result in the yellowing of the plants and weakening of their growth potential[24].
Therefore, in this study, apple self-rooted rootstock M9-T337 were used as materials to investigate the effect of AMF inoculation on the improvement of leaf physiological characteristics of apple rootstock M9-T337 under saline-alkaline stress, to provide a theoretical basis and technical support for saline-alkaline tolerant cultivation of apple. Meanwhile, it is of great scientific significance and practical value to further clarify that AMF improves the saline-alkaline resistance of rootstock M9-T337 and to reveal the intrinsic mechanisms by which AMF promotes the growth and saline-alkaline tolerance of apple rootstocks. This will lay a theoretical foundation for the efficient cultivation of apples and the development of stress-resistant varieties, which is crucial for the sustainable production of apples in saline-alkaline environments.
-
The test material was the apple M9-T337 self-rooted rootstock. The test strain, Funneliformis mosseae (AMF), was provided by the Bank of Glomeromycota in China (BGC HEBO7B). The inoculum consisted of spores, mycelium, root systems, and root substrates, which were prepared for use after three months of expanded propagation by red Clover (Trifolium pratense L.).
Experimental treatment
-
The experiment was conducted in February 2023 in a rain shelter at Gansu Agricultural University, Gansu, China (36°1' to 37°9' N, 106°21' to 107°44' E). A total of 360 uniform and disease-free one-year-old apple rootstock M9-T337 were selected. They were transferred into 360 pots (25 cm in diameter and 38 cm in depth) containing 3.5 kg of substrate (river sand : soil = 2:1) and then watered. During transplanting, rootstocks were divided into two groups: AMF-inoculated and non-AMF-inoculated. The AMF-inoculated group was transplanted by mixing it evenly in the substrate and inoculating each pot with 10 g of AMF (52 spores per gram). The group without AMF treatment was planted with substrate only. Saline-alkali treatment was carried out after 20 d of incubation. According to a previous study by the project team[25], saline-alkali stress was carried out by root irrigation with the appropriate concentration of saline-alkali solution (0, 50, 100, and 150 mM NaCl + NaHCO3, NaCl : NaHCO3 = 1:1) in each pot. Irrigation was carried out every 6 d, four times. Each watering was 500 mL. To avoid the phenomenon of saline-alkali shock during saline stress, the first stress was watered with saline-alkali solution in batches, each time at a concentration of 50 mM, once a day, until the concentration of different saline-alkali treatments was reached.
The experiment included eight treatments: (1) Fresh water irrigation (N0), (2) 50 mM NaCl + NaHCO3 (N50), (3) 100 mM NaCl + NaHCO3 (N100), (4) 150 mM NaCl + NaHCO3 (N150), (5) Fresh water irrigation + AMF (M0), (6) 50 mM NaCl + NaHCO3 + AMF (M50), (7) 100 mM NaCl + NaHCO3 + AMF (M100), (8) 150 mM NaCl + NaHCO3 + AMF (M150). Each treatment contained three replicates, with each replicate containing 15 rootstocks. To minimize environmental variations and bias, a randomized block design was used to arrange the pots. Samples were taken 30 d after the onset of saline-alkali stress, and relevant indexes were measured.
Growth indexes
-
One rootstock was randomly selected for each treatment and placed in an environment with a black cloth background for taking photos to observe the phenotype of rootstock M9-T337. Five rootstocks per treatment were randomly selected to determine the growth index. Leaf length, leaf width, leaf thickness, leaf area, and leaf circumference were measured using a leaf area meter and the aspect ratio was calculated.
Observation of AMF colonization ratio
-
Determination of mycorrhizal colonization ratio by trichothecene blue staining-cross-hatching method[26]. The roots of the plants were cut into 1 cm root segments, and 50 root segments were taken from the root system of each rootstock. The root samples were processed for transparency, acidification, staining, decolorization, and restaining.
Mycorrhizal colonization ratio = (Number of root segments with mycorrhizal colonization/Total root segments measured) × 100%.
Observation of leaf microstructure
-
The leaf tissue microstructure was observed according to the method described by Hu et al.[26]. Functional leaves from the same node at the top of M9-T337 plants were collected and thoroughly rinsed with distilled water. Small leaf pieces of 0.25 cm² (0.5 cm × 0.5 cm), containing the main vein and adjacent areas, were cut with a razor blade and placed in FAA (formalin-acetic acid-alcohol) fixative for fixation. The samples were then vacuum-pumped and removed from the fixative. The sectioning process included rinsing, dehydration, clearing, wax dipping, embedding, trimming, slicing, patching, spreading, and sealing. Double staining was performed using safranine and solid green. The sections were sealed with neutral gum and stored. The section thickness was maintained at 10 μm. The leaf thickness, upper and lower epidermal thickness, palisade tissue thickness, and spongy tissue thickness were observed, photographed, and measured within the leaf mesophyll tissue structure using a positive-inverted integrated fluorescence microscope (Revolve RVL-100-G, ECHO, USA). For each treatment, five sections were observed and for each section, three fields of view were randomly selected and averaged. The following indicators were calculated:
The leaf tissue structure compactness (CTR) = (Thickness of palisade tissue/Total thickness of blade) × 100%
The leaf tissue structure sparseness (CR) = (Sponge tissue thickness/Total leaf thickness) × 100%
Leaf stomatal observation
-
The stomatal characteristics were observed following the method described in the study by Song et al.[27]. First, the leaf surface was rinsed with distilled water and then a middle portion of the leaf was excised and immersed in a 2.5% glutaraldehyde fixative. The sample was subjected to vacuum infiltration and stored at 4 °C for 2 to 4 d. Afterward, the samples were washed with phosphate buffer three times, with each wash lasting 15 min. They were then dehydrated through a graded ethanol series: 30%, 50%, 70%, 80%, 90%, and 95%, with each step also lasting 15 min. Subsequently, the samples were transferred to 100% tertiary butyl alcohol for two changes, each lasting 20 min. Once fully dehydrated, the samples were dried, affixed to a scanning electron microscope (SEM) stage, and coated with a metal film using a vacuum ion sputtering apparatus. Finally, the samples were observed and photographed under the SEM. Measurement of stomatal width, stomatal length, guard cell length, and guard cell width with Image-J software. Stomatal length (Lp), stomatal width (Wp), guard cell length (Lg), guard cell width (Wg), stomatal density (SD), stomatal size (SS), stomatal aperture (Sa), and maximum stomatal aperture (Gsmax) were calculated using the following formulas:
Sa=π×Lp×Wp/4 SS=π×Lg×Wg/4−Sa Gsmax=d×αmax×SD/v×(Lp+π/2×√αmax/π) where π is taken as 3.14, d is the diffusivity of water in air (24.6 × 10−6 m2·s−1, 25 °C), αmax is the mean maximum stomatal area, v is the molar volume of air (24.4 × 10−3 m3·mol−1, 25 °C and 101.3 kPa).
Chlorophyll fluorescence parameters
-
The fluorescence parameters were determined by IMAGING-PAM chlorophyll fluorescence imager[28]. After 30 min dark treatment, the fluorescence parameters initial fluorescence (F0), maximum fluorescence (Fm), quantum yield of unregulated energy dissipation (Y(N0)), and photochemical quenching coefficient (qP) were analyzed by Imaging Win Geg Esoft-ware software.
Endogenous hormone
-
The leaf endogenous hormone content was determined according to the method described by Yan et al.[29]. First, 2 g of M9-T337 leaves were weighed and placed in a mortar and pestle. The leaves were quickly powdered by adding liquid nitrogen and then washed three times with 10 mL of 80% methanol (prepared with ultrapure water) in a 15 mL centrifuge tube. The powder was extracted in a refrigerator at 4 °C for 24 h, during which it was thoroughly mixed and shaken every hour. After extraction, the mixture was centrifuged for 15 min. The supernatant was transferred into a new centrifuge tube and concentrated using a rotary evaporator at 40 °C to remove methanol, yielding a concentrate of approximately 2 mL. The walls of the evaporation flask were then rinsed with 50% methanol and the volume was adjusted to 10 mL. The concentrate was filtered through a 0.22 μm organic membrane using a disposable syringe into a 1.5 mL centrifuge tube and the volume was adjusted again to 10 mL. The extract was stored in the refrigerator at −20 °C in the dark for the determination of zeatin (ZT), zeatin riboside (TZR), gibberellin (GA3), indoleacetic acid (IAA), and salicylic acid (SA) contents. The extract solution for gibberellin was prepared with acetonitrile, following the same extraction procedure as described above.
Separation was carried out using an Agilent 1100 series liquid chromatograph equipped with a VWD detector and an Extend-C18 column (4.6 mm × 250 mm, 5 μm). The mobile phase consisted of methanol and 0.1% phosphoric acid (methanol : phosphoric acid = 1:9) at a flow rate of 1.0 mL/min and a detection wavelength of 254 nm. The endogenous hormone content of the samples was determined using the external standard method.
Na+ and K+ content
-
Na+ and K+ content were measured using the flame photometric method. Leaves were dried, ground, and accurately weighed into 5 g leaf powder samples. These samples were then digested with H2SO4 and H2O2 at 220 °C. The Na+ and K+ contents were measured using a flame photometer (M410, Sherwood Scientific, Cambridge, UK), and the Na+/K+ ratios were calculated.
Data processing
-
Data processing was performed using Microsoft Office Excel 2019 and the graphs were plotted using Origin 2022 software. Statistical analysis was carried out using the IBM SPSS Statistics 25 program (SPSS Inc., Chicago, IL, USA). An analysis of variance (ANOVA) was used to compare mean values between samplings. The Student's t-test was used to determine differences between treatments.
-
As can be seen in Fig. 1, all apple rootstocks M9-T337 inoculated treatments could develop a symbiotic relationship with AMF. The Mycorrhizal colonization ratio of all treatments showed a decreasing trend with the increase of saline-alkali concentration, which was 41.23%, 39.36%, 35.24%, and 30.12%, respectively. Mycorrhizal colonization ratios were significantly different between the control and different saline-alkali treatments.
Figure 1.
Effects of saline-alkali stress on the mycorrhizal infestation rate of apple M9-T337 rootstock by arbuscular mycorrhizal. (M0) fresh water irrigation + AMF; (M50) 50 mM NaCl + NaHCO3 + AMF; (M100) 100 mM NaCl + NaHCO3 + AMF; (M150) 150 mM NaCl + NaHCO3 + AMF). * Represents a significant correlation at the 0.0.5 level; ** represents a highly significant correlation at the 0.01 level (Student's t-test).
AMF can boost the leaf growth parameters of M9-T337 under saline-alkali stress
-
As can be seen from Fig. 2, the growth of apple rootstock M9-T337 leaves was inhibited under saline-alkali stress. However, the inoculation with AMF promoted the growth of rootstock M9-T337. Compared to the N0 treatment, leaf area, leaf thickness, leaf width, leaf length, and leaf perimeter were significantly reduced under various saline-alkali concentrations, with decreases ranging from 32.66% to 34.15%, 4.01% to 38.97%, 3.22% to 18.33%, 14.37% to 33.34%, and 22.96% to 33.76%, respectively. Inoculation with AMF under saline-alkali stress conditions resulted in increased leaf area, leaf thickness, leaf width, leaf length and leaf perimeter in rootstock M9-T337, with the highest values observed in the M100 treatment. These increases were 1.25, 1.71, 1.03, 1.25, and 1.27 times greater than those in the N100 treatment, respectively (Table 1).
Figure 2.
Effects of AMF on phenotype 0f M9-T337 leaves under saline-alkali stress. (M0) Fresh water irrigation + AMF; (N0) fresh water irrigation; (M50) 50 mM NaCl + NaHCO3 + AMF; (N50) 50 mM NaCl + NaHCO3; (M100) 100 mM NaCl + NaHCO3 + AMF; (N100) 100 mM NaCl + NaHCO3; (M150) 150 mM NaCl + NaHCO3 + AMF); (N150) 150 mM NaCl + NaHCO3.
Table 1. Effects of AMF on phenotype 0f M9-T337 leaves under saline-alkali stress.
Treatments Blade thickness (μm) Leaf width (μm) Leaf length (μm) Leaf area (μm) Blade perimeter (μm) Length/width M0 298.30 ± 9.87a 52.06 ± 1.88a 47.70 ± 2.22a 1907.73 ± 106.87a 652.32 ± 71.19a 0.91 ± 0.01ab N0 291.80 ± 10.14a 45.62 ± 3.56b 47.12 ± 2.67a 1433.16 ± 43.85b 529.07 ± 20.85b 1.05 ± 0.08a M50 300.10 ± 50.92a 46.47 ± 3.05ab 41.26 ± 1.33ab 1280.90 ± 206.76a 506.16 ± 11.02a 0.89 ± 0.01a N50 280.10 ± 2.93b 44.15 ± 1.46b 40.35 ± 0.83b 956.87 ± 7.74b 407.62 ± 7.42b 0.91 ± 0.04a M100 309.30 ± 28.74a 42.84 ± 2.19bc 39.27 ± 1.16a 1210.65 ± 39.82a 420.23 ± 30.11a 0.94 ± 0.07a N100 181.30 ± 14.28b 41.53 ± 0.47bcd 31.41 ± 1.82b 965.13 ± 61.19b 330.13 ± 21.95b 0.64 ± 0.01b M150 183.6 ± 18.81a 36.25 ± 1.63ab 45.38 ± 1.77a 943.76 ± 32.43ab 459.74 ± 51.02a 1.25 ± 0.17a N150 178.10 ± 12.39a 37.26 ± 3.44a 35.78 ± 2.38b 971.73 ± 26.19a 329.27 ± 20.34b 0.96 ± 0.13b (M0) Fresh water irrigation + AMF; (N0) fresh water irrigation; (M50) 50 mM NaCl + NaHCO3 + AMF; (N50) 50 mM NaCl + NaHCO3; (M100) 100 mM NaCl + NaHCO3 + AMF; (N100) 100 mM NaCl + NaHCO3; (M150) 150 mM NaCl + NaHCO3 + AMF; (N150) 150 mM NaCl + NaHCO3. Different lowercase letters in the same column indicate significant difference at the 0.05 level. Effects of AMF on the microstructure of M9-T337 leaves under saline-alkali stress
-
Figure 3 shows the effect of AMF inoculation on the microstructure of M9-T337 leaf cells under saline-alkali stress. Under saline-alkali stress, the microstructure of M9-T337 leaf cells was damaged and both its palisade and spongy tissues underwent obvious changes, which were that the thickness of the palisade tissues was significantly reduced and arranged loosely and irregularly, and the cells of the spongy tissues were made smaller and the cellular gaps were enlarged. Compared with the N0 treatment, the leaf thickness (LT), palisade tissue (PT), spongy tissue (ST), upper epidermal thickness (UE), lower epidermal thickness (LE), leaf tissue tightness (CTR), and leaf tissue looseness (CR) of M9-T337 leaves were significantly reduced under different concentrations of saline-alkali stress. In contrast, the thickness of the palisade tissue of M9-T337 leaves increased and became more neatly arranged after inoculation with AMF, the cells of spongy tissues became larger and the cell gaps were reduced. Additionally, all the indexes of the leaves were increased to different degrees, with the most complete leaf microstructure under M100 treatment (p < 0.05), which increased 60.09%, 51.88%, 84.45%, 74.56%, 32.40%, 5.20%, 14.60%, respectively, compared with N100 treatment (Table 2).
Figure 3.
Effect of AMF on the microstructure of M9-T337 leaves under saline-alkali stress. (N0) Fresh water irrigation; (M0) fresh water irrigation + AMF; (N50) 50 mM NaCl + NaHCO3; (M50) 50 mM NaCl + NaHCO3 + AMF; (N100) 100 mM NaCl + NaHCO3; (M100) 100 mM NaCl + NaHCO3 + AMF; (N150) 150 mM NaCl + NaHCO3; (M150) 150 mM NaCl + NaHCO3 + AMF.
Table 2. Effect of AMF on the microstructure of M9-T337 leaves under saline-alkali stress.
Treatments Thickness of leaf (μm) Upper skin thickness (μm) Lower skin thickness (μm) Thickness of palisade (μm) Thickness of spongy (μm) Cell tightness rate (CTR,%) Scattered rate (CR,%) PT/ST M0 275.30 ± 9.97a 16.10 ± 0.92a 16.45 ± 3.61a 135.80 ± 14.53a 122.30 ± 8.20a 44.02 ± 1.84a 44.42 ± 2.98ab 0.89 ± 0.03n N0 204.30 ± 10.32b 12.90 ± 0.99b 7.60 ± 1.27b 82.95 ± 3.75b 93.55 ± 6.86b 40.60 ± 6.29b 45.79 ± 2.11a 1.14 ± 0.07a M50 304.70 ± 52.96a 22.50 ± 0.42a 15.15 ± 1.06a 162.90 ± 25.57a 116.40 ± 7.50a 53.46 ± 2.57b 38.20 ± 2.46a 1.78 ± 0.03a N50 281.10 ± 2.97b 21.20 ± 3.96ab 10.90 ± 0.57b 159.07 ± 6.33a 87.57 ± 2.64b 56.59 ± 9.67a 31.15 ± 1.21b 1.28 ± 0.05b M100 305.30 ± 29.41a 19.90 ± 1.84a 11.85 ± 3.61a 135.10 ± 18.24a 171.80 ± 17.68a 44.25 ± 6.08a 56.27 ± 8.03a 0.92 ± 0.13a N100 190.70 ± 14.35b 11.40 ± 3.54b 8.95 ± 1.20b 83.95 ± 5.44b 93.65 ± 19.73b 44.02 ± 5.98a 49.11 ± 10.34b 0.83 ± 0.07b M150 184.30 ± 18.88a 10.67 ± 3.33a 8.85 ± 1.06a 104.17 ± 9.68a 76.77 ± 7.32a 56.52 ± 5.38b 41.65 ± 4.11a 1.24 ± 0.01a N150 181.40 ± 12.94a 11.05 ± 2.47a 7.45 ± 1.06b 97.90 ± 9.76ab 73.40 ± 0.71a 53.97 ± 14.46b 40.46 ± 0.39ab 1.16 ± 0.03a (M0) Fresh water irrigation + AMF; (N0) fresh water irrigation; (M50) 50 mM NaCl + NaHCO3 + AMF; (N50) 50 mM NaCl + NaHCO3; (M100) 100 mM NaCl + NaHCO3 + AMF; (N100) 100 mM NaCl + NaHCO3; (M150) 150 mM NaCl + NaHCO3 + AMF); (N150) 150 mM NaCl + NaHCO3. Different lower case letters in the same column indicate significant difference at the 0.05 level. Effect of AMF on chlorophyll fluorescence parameters of M9-T337 leaves under saline-alkali stress
-
Figure 4 represents the effect of AMF inoculation and no AMF on chlorophyll fluorescence parameters of M9-T337 leaves under saline-alkali stress. There was an overall decreasing trend in Fv/Fm, Fm, F0, Y(N0), and qP of Rootstock M9-T337s leaves with increasing saline-alkali concentration. Fv/Fm, Fm, F0, Y(N0), and qP of leaves under different concentrations of saline-alkali treatments showed a significant decrease compared to N0 treatment, ranging from 25.58% to 39.53%, 7.85% to 22.51%, 16.20% to 40.14%, 13.45% to 23.47%, and 6.42% to 13.86% of N0 treatment, respectively. Inoculation of AMF treatments based on saline-alkali stress all resulted in an increasing trend in Fv/Fm, Fm, F0, Y(N0), and qP in the leaves of rootstock M9-T337, with the greatest degree of increase in the M100 treatment, which was 57.97%, 37.74%, 37.50%, 11.48%, and 16.30% of the N100 treatment, respectively.
Figure 4.
Effect of AMF on fluorescence parameter of M9-T337 leaves under saline-alkali stress. NM (Inoculation with AMF); AM (Uninoculation with AMF). (a) Chlorophyll fluorescence imaging. (b) Fv/Fm, PSIImaximal photochemical efficiency. (c) Fm, maximum fluorescence. (d) F0, initial fluorescence. (d) Y(N0), quantum yield of unregulated energy dissipation. (f) qP, photochemical quenching coefficient. * Represents a significant correlation at the 0.0.5 level; ** represents a highly significant correlation at the 0.01 level (Student's t-test).
Effect of AMF on stomatal morphology and structure of M9-T337 leaves under saline-alkali stress
-
As depicted in Fig. 5, the stomata of M9-T337 leaves under different saline-alkali stress treatments showed different degrees of closure. However, after inoculation with AMF, the stomata of leaves of all treatments showed different degrees of opening. The effect of change was most significant under M100 treatment, as evidenced by the Lp, Wp, Lg, Wg, SD, SS, Sa, and Gsmax of Rootstock M9-T337s leaves under M100 treatment increased by 45.12%, 139.76%, 22.00%, 22.86%, 62.08%, 44.38%, 247.98%, and 127.40%, respectively, compared with N100 treatment (Table 3).
Figure 5.
Effect of AMF on stomatal morphology of M9-T337 leaves under saline-alkali stress. (N0) Fresh water irrigation; (M0) fresh water irrigation + AMF; (N50) 50 mM NaCl + NaHCO3; (M50) 50 mM NaCl + NaHCO3 + AMF; (N100) 100 mM NaCl + NaHCO3; (M100) 100 mM NaCl + NaHCO3 + AMF; (N150) 150 mM NaCl + NaHCO3; (M150) 150 mM NaCl + NaHCO3 + AMF.
Table 3. Effect of AMF on stomatal morphology of M9-T337 leaves under saline-alkali stress.
Treaments Lp (μm) Wp (μm) Lg (μm) Wg (μm) SD (mm2) SS (μm2) Sa (μm2) Gsmax (μm3) N0 14.99 ± 0.32a 2.19 ± 0.44a 25.94 ± 0.98a 18.88 ± 0.67a 218.72 ± 2.44b 358.68 ± 43.54ab 25.77 ± 6.10a 1.35 ± 0.87b M0 15.03 ± 0.33a 2.27 ± 0.48a 25.99 ± 1.00a 19.43 ± 0.72a 274.93 ± 9.31a 369.63 ± 64.56a 26.78 ± 6.67a 1.7 ± 0.96a N50 11.70 ± 0.44a 1.11 ± 0.09ab 21.43 ± 0.10ab 15.32 ± 0.46ab 201.38 ± 9.11ab 247.53 ± 38.23b 10.19 ± 4.13a 0.99 ± 0.67ab M50 12.1 ± 0.16a 1.36 ± 0.05a 22.76 ± 0.20a 16.83 ± 0.45a 218.94 ± 7.67a 287.78 ± 35.63a 12.92 ± 4.51a 1.12 ± 0.7a N100 10.24 ± 0.54b 0.83 ± 0.09b 20.68 ± 0.68b 15.18 ± 0.09b 166.58 ± 9.1b 239.76 ± 29.79b 6.67 ± 2.89b 0.73 ± 0.54b M100 14.86 ± 0.61a 1.99 ± 0.21a 25.23 ± 0.22a 18.65 ± 0.75a 270.33 ± 9.14a 346.16 ± 54.44a 23.21 ± 4.99a 1.66 ± 0.99a N150 9.16 ± 0.81b 0.60 ± 0.72ab 19.84 ± 0.66ab 14.21 ± 1.33ab 121,43 ± 9.49b 216.99 ± 25.59ab 4.31 ± 2.23ab 0.49 ± 0.42b M150 11.77 ± 0.69a 0.80 ± 0.07a 20.49 ± 0.68a 15.21 ± 0.10a 206.85 ± 7.23a 237.26 ± 30.04a 7.39 ± 3.77a 1.03 ± 0.52a (M0) Fresh water irrigation + AMF; (N0) fresh water irrigation; (M50) 50 mM NaCl + NaHCO3 + AMF; (N50) 50 mM NaCl + NaHCO3; (M100) 100 mM NaCl + NaHCO3 + AMF; (N100) 100 mM NaCl + NaHCO3; (M150) 150 mM NaCl + NaHCO3 + AMF); (N150) 150 mM NaCl + NaHCO3. Different lower case letters in the same column indicate significant difference at the 0.05 level. Effect of AMF on endogenous hormone contents of M9-T337 leaves under saline-alkali stress
-
As described in Fig. 6, the ZT, TZR, GA3, IAA, and SA contents of rootstock M9-T337 leaves were decreased, while the ABA content was increased under saline-alkali stress treatments. However, inoculation with AMF increased ZT, TZR, GA3, IAA, and SA contents and decreased ABA in rootstock leaves. Compared to the N0 treatment, the contents of ZT, TZR, GA3, IAA, and SA in leaves under different concentrations of saline-alkali treatments showed a significant decrease ranging from 35.96% to 71.46%, 18.43% to 50.04%, 18.72% to 38.56%, 15.88% to 24.61%, and 12.71% to 37.81%, respectively. While ABA content showed an increasing trend, which was 7.62% to 21.01% of N0 treatment. Inoculation of AMF treatments based on saline-alkali stress resulted in an increasing trend of ZT, TZR, GA3, IAA, and SA contents in the leaves of rootstock M9-T337, with the greatest degree of increase in the M100 treatment, which was 71.30%, 62.35%, 41.23%, 41.68%, and 49.67% of that in the N100 treatment, respectively. On the contrary, inoculation with AMF treatments reduced the increasing trend of ABA content in the leaves of rootstock M9-T337, with the most significant change in M100, which was 44.43% of the N100 treatment.
Figure 6.
Effect of AMF on endogenous hormone of M9-T337 leaves under saline-alkali stress. NM (Inoculation with AMF); AM (Uninoculation AMF). (a) Zeatin content, (b) maize kernel riboside content, (c) gibberellin content, (d) indole acetic acid content, (e) abscisic acid content, (f) salicylic acid content. * Represents a significant correlation at the 0.0.5 level; ** represents a highly significant correlation at the 0.01 level (Student's t-test).
Effects of AMF on Na+ and K+ contents of M9-T337 leaves under saline-alkali stress
-
As depicted in Fig. 7, both Na+ content and the Na+/K+ ratio in Rootstock M9-T337s leaves showed a gradual increase with rising saline-alkali concentrations, ranging from 2.13 to 4.48 and 2.20 to 5.98 times that of the N0 treatment, respectively. Conversely, the K+ content in the leaves exhibited a decreasing trend with increasing saline-alkali concentrations, ranging from only 3.42% to 25.34% of the N0 treatment. Inoculation of AMF under saline-alkali stress resulted in various reductions in Na+ content and the Na+/K+ ratio in the leaves, while the K+ content increased to varying extents. The most significant changes were observed under the M100 treatment. Specifically, the Na+ content and the Na+/K+ ratio decreased by 24.18% and 40.71%, respectively, compared to the N100 treatment, while the K+ content increased by 1.28 times compared to the N100 treatment.
Figure 7.
Effect of AMF on changes of sodium and potassium contents of M9-T337 leaves under saline-alkali stress. NM (Inoculation with AMF); AM (Uninoculation AMF). (a) Leaf Na+ content, (b) leaf K+ content, (c) leaf Na+ /K+content. * Represents a significant correlation at the 0.0.5 level; ** represents a highly significant correlation at the 0.01 level (Student's t-test).
Effects of AMF on soil Na+ and K+ contents under saline-alkali stress
-
Figure 8 illustrates the significant effect of AMF on the ionic content of soil under different saline-alkali concentration treatments. Compared to the N0 treatment, both Na+ content and the Na+/K+ ratio in the soil showed a significant increasing trend under various saline-alkali treatments, increasing by 2.60 to 5.67 times and 2.92 to 10.00 times, respectively. Conversely, the K+ content in the soil exhibited a decreasing trend, ranging from 11.02% to 43.31% of the N0 treatment levels. The inoculation of AMF under saline-alkali stress conditions resulted in a decreasing trend of Na+ content and the Na+/K+ ratio in the soil, with the most pronounced decrease observed under the M100 treatment, reducing to 44.16% and 36.05% of the N100 treatment, respectively. Meanwhile, the K+ content in the soil showed a significant increasing trend following AMF inoculation, with the most notable increase observed in the M100 treatment, reaching 63.02% of the N100 treatment level.
Figure 8.
Effect of AMF on changes of sodium and potassium contents of soil under saline-alkalii stress. NM (Inoculation with AMF); AM (Uninoculation AMF). (a) Soil Na+ content, (b) soil K+ content, (c) soil Na+/K+content. * Represents a significant correlation at the 0.0.5 level; ** represents a highly significant correlation at the 0.01 level (Student's t-test).
Comprehensive evaluation of the physiological effects of AMF on apple rootstock M9-T337 under saline-alkali stress
Correlation analysis
-
The analysis of correlation was carried out on 28 physio-logical indicators of the leaves of rootstock M9-T337 after treatment. As illustrated in Fig. 9, the findings revealed a highly significantly positive correlation (p < 0.01) between F0 of the leaves of rootstock M9-T337 and Fm, qP, Y(N0), K+, Wp, Lg, and Wg. Furthermore, it was a significant positive correlation correlation between LT, LE, ZT, IAA, SA, Lp, Sa, SS, SD, and Gsmax (p < 0.05). Moreover, F0 showed a very significantly negative correlation (p < 0.01) with Na+, PS/ST, CR, and ABA.
Figure 9.
Correlation analysis of AMF on physiological indexes of M9-T337 rootstock under saline-alkali stress. * Represents a significant correlation at the 0.0.5 level; ** represents a highly significant correlation at the 0.01 level.
Principal component analysis
-
To comprehensively assess the physiological response of AMF to different saline-alkali concentrations in the leaves of rootstock M9-T337, 28 physiological indicators were subjected to principal component analysis (PCA). Two principal components with eigenvalues greater than 1 were extracted, which were 16.656 and 4.917, respectively. The first and second principal components accounted for 86.711% and 9.732% of the variance, respectively. Furthermore, the cumulative variance contribution rate reached 96.443% (Table 4). All meet the analysis requirements.
Table 4. Total variance explained.
Idex Eigen value Proportion of variance (%) Cumulative variance (%) PC1 16.656 86.711 86.711 PC2 4.917 9.732 96.443 PC1−2 respectively principal component 1−2. Comprehensive scores ranking
-
The comprehensive scores were ranked based on the indicator data represented by the two principal components. F1 and F2 represent the first and second principal components, respectively. The comprehensive score (F) was calculated by summing up the product of the scores for each principal component and the corresponding variance contribution rate. In other words, F = F1 × 86.711% + F2 × 9.732%. As presented in Table 5, the comprehensive scores of M9-T337 leaves under various treatments were −1.041496 (N0), 1.220719 (M0), −0.068310 (N50), 0.450354 (M50), −0.889780 (N100), 1.102925 (M100), −1.256300 (N150), and −0.734030 (M150), respectively. Consequently, the ranking of the effect scores of AMF on the physiological characteristics of apple rootstock M9-T337 under saline-alkali stress was as follows: M0 > M100 > N0 > M50 > N50 > M150 > N100 > N150.
Table 5. Comprehensive score ranking of M9-T337 seedling leaves under different treatments.
Treatments Principal component score Comprehensive
score (F)Comprehensive
score rankingPC1 (F1) PC2 (F2) N0 1.04054 1.43068 1.041496 3 M0 1.26879 1.23858 1.220719 1 N50 −0.2474 1.50243 −0.068310 5 M50 0.38632 1.18549 0.450354 4 N100 −0.95254 −0.65578 −0.889780 7 M100 1.22317 0.43467 1.102925 2 N150 −1.40833 −0.3609 −1.256300 8 M150 −0.81908 −0.24448 −0.734030 6 (M0) Fresh water irrigation + AMF; (N0) fresh water irrigation; (M50) 50 mM NaCl + NaHCO3 + AMF; (N50) 50 mM NaCl + NaHCO3; (M100) 100 mM NaCl + NaHCO3 + AMF; (N100) 100 mM NaCl + NaHCO3; (M150) 150 mM NaCl + NaHCO3 + AMF); (N150) 150 mM NaCl + NaHCO3. Different lower case letters in the same column indicate significant difference at the 0.05 level. -
Under abiotic stress, plants undergo a series of morphological changes, particularly in the leaves[30]. Plants produce a series of stress responses under saline-alkali stress, which are accompanied by various morphological and physiological changes, including alterations in plant characteristics, leaf photosynthetic capacity, phytohormone content, and leaf microstructure[31].
A typical morphological response of plant leaves to saline-alkali stress includes leaf thickening, increased leaf area, and heightened leaf succulence. Previous studies have shown a decreasing trend in leaf length, leaf area, and leaf weight of Pyrus betulaefolia Bunge leaves with increasing levels of saline-alkali stress[32]. In this study, the leaf area, leaf perimeter, leaf width and leaf thickness of apple rootstock M9-T337 significantly decreased with rising saline-alkali concentrations. This phenomenon may be attributable to an osmotic pressure imbalance caused by high saline-alkali levels in the soil, which hindered plant cells from absorbing water properly, thus limiting leaf expansion and growth[33]. Furthermore, high saline-alkali concentrations may directly damage organelles such as chloroplasts, affecting photosynthesis efficiency, and reducing the production of energy and organic matter[34]. In addition, saline-alkali environments can hinder the plant's ability to absorb essential nutrients, causing nutrient deficiencies. These deficiencies, in turn, disrupt physiological and biochemical processes such as respiration, enzyme activity, and hormone balance, collectively inhibiting normal leaf development[35]. Conversely, the leaf area, leaf perimeter, and leaf width of rootstock M9-T337 leaves were improved by inoculation with AMF treatments, with the best mitigation observed under the M100 treatment. This improvement may be attributed to AMF enhancing the root system surface area of rootstock M9-T337 under saline-alkali stress, thereby improving water and nutrient absorption[36]. Furthermore, AMF may assist the plant in regulating osmotic pressure both inside and outside the cells, reducing the cellular toxicity of saline-alkali conditions, improving saline-alkali tolerance, and promoting cell division and elongation by stimulating the production of growth hormones[14].
Leaf microstructure plays a pivotal role in the physiological functioning of plants and their ability to adapt to the environment, and it directly affects photosynthesis efficiency and facilitates energy conversion and organic matter synthesis through the distribution and morphology of chloroplasts[37]. Previous studies have shown that the upper and lower epidermis of plant leaves become thinner and the layers of palisade and spongy tissues, cell diameters, and cell gaps become smaller, resulting in a reduction in leaf pulp thickness under saline-alkali stress[38]. Our experiment showed that leaf thickness, the upper and lower epidermal thickness, and the thickness of palisade and spongy tissues were reduced in rootstock M9-T337 under saline-alkali stress. This may be due to the reduction of water content in rootstock M9-T337 under saline-alkali stress, which affects the integrity of cellular structures and inhibits both the water metabolism of the leaves and the growth metabolism of the cells[39]. Additionally, the blockage of cell division and elongation affects the development of leaf tissues[40]. However, all the tissue structures of the leaves increased to different degrees after inoculation with AMF treatments. This may be due to AMF increasing the root system surface area of rootstock M9-T337 under saline-alkali stress, improving water and nutrient uptake efficiency, possibly regulating endogenous hormone levels and enhancing photosynthetic efficiency[41].
Chlorophyll fluorescence parameters, as probes of photosynthetic induction, can rapidly and non-destructively detect the response mechanism of the PSII photosystem to adversity, indirectly reflecting the light energy uptake and transfer of photosynthesis, as well as the process of photosynthetically induced energy utilization and dissipation[42]. Some studies found that chlorophyll fluorescence parameters tend to decrease sharply with increasing saline-alkali stress[43]. One study on soybean indicated that the photosynthetic indexes of soybean leaves significantly reduced under different concentrations of saline-alkali stresses[44]. In this experiment, Fv/Fm, F0, Fm, qP, and Y(NO) of M9-T337 leaves showed a decreasing trend with increasing saline-alkali stress. The decrease in Fv/Fm may be because the plants were undergoing photoinhibition under saline-alkali stress[45]. The decrease in F0 value may be because saline-alkali stress damaged the PSI reaction centers in the leaves of M9-T337, which inhibited the normal photosynthetic reactions of the plants[46]. The decrease in Fm indicated that saline-alkali stress inhibits the electron transport chain during the photosynthetic reaction, reducing the amount of NADPH and ATP assimilated by the cell and affecting carbon fixation and assimilation processes. The decrease in qP indicated that photochemical energy transfer was inhibited under saline-alkali stress[47]. Collectively, these findings demonstrated that saline-alkali stress causes damage to the photosynthetic system of the plant, leading to a decrease in the rate of electron transfer during photosynthesis, which in turn affects normal plant growth. These results align with the study by Yang et al. on Robinia pseudoacacia[48]. After inoculation with AMF treatments, the parameters Fv/Fm, F0, Fm, qP, and Y(NO) of rootstock M9-T337 leaves were increased to varying degrees, enabling the plants to photosynthesize normally. This indicates that AMF application can reduce the inhibition of saline-alkali stress on the growth of rootstock M9-T337 by increasing fluorescence parameters and maintaining normal photosynthesis[49]. However, the specific mechanism of photochemical efficiency of AMF in response to saline-alkali stress warrants further investigation.
There is a wide variety of endogenous hormones in plants, and there are synergistic or antagonistic effects between different hormones, which jointly promote plant acclimatization to adversity stress[50]. Phytohormones play crucial roles in processes such as plant growth, development, and stress response to the environment[51]. Numerous studies have shown that plant hormones regulate plant growth and development not only under normal conditions but also under various environmental stresses, such as saline-alkali stress[52]. A study by Liu et al. reported that ethylene and ABA contents were elevated in apple rootstock under saline-alkali stress, while IAA, GA3, and CTK contents were decreased[53]. One study found that under high salt stress, the content of GA3 decreased, causing growth inhibition in plants[54]. In this study, the contents of ZT, TZR, IAA, GA3, and SA in rootstock M9-T337 decreased to varying degrees with increasing saline-alkali stress concentration, while ABA content significantly increased. This may be because saline-alkali stress inhibited the synthesis of growth-promoting hormones such as IAA and GA3. Meanwhile, rootstock M9-T337 may regulate their endogenous hormone levels to adapt to osmotic pressure changes and enhance stress tolerance[55,56]. The increase in ABA content may be related to improved water use efficiency, enhanced oxidative stress defense, and regulated stomatal movement to reduce water evaporation. After inoculation with AMF treatments, the contents of ZT, TZR, IAA, GA3, and SA in rootstock M9-T337 increased, while ABA content decreased. This may be because AMF improved nutrient and water uptake and promoted the synthesis of growth hormones, thus increasing the levels of ZT, TZR, IAA, GA3, and SA[57]. The symbiotic relationship formed between AMF and the root system may enhance root health and contribute to more efficient soil resource utilization, thereby affecting hormonal balance[58]. These results indicated that AMF application can alleviate saline-alkali stress in rootstock M9-T337 by increasing the content of endogenous hormones such as ZT, TZR, IAA, GA3, and SA and decreasing the content of ABA.
Under saline-alkali stress, Na+ concentrations increase in both soil and leaves and high concentrations of Na+ can negatively affect plant growth by inhibiting water uptake, destabilizing cell membranes, and interfering with normal metabolic processes[50]. Additionally, there was competition between Na+ and other ions such as Ca2+ and Mg2+ in the soil, which affected the uptake and utilization of these ions by plants[59].
In contrast to Na+, saline-alkali stress usually leads to a decrease in soil K+ content. K+ is an essential nutrient for plants, significantly influencing its growth and development. Under saline-alkali stress, K+ in the soil may be replaced by Na+ or rendered immobilized, hindering its effective uptake by plants. Moreover, high concentrations of Na+ may inhibit active K+ uptake processes in plant roots[60]. AMF can influence the distribution and availability of ions in the soil. The mycelial network of AMF absorbs and immobilizes Na+ in the soil, thereby mitigating and reducing its toxicity to plants[61]. Numerous studies have found that AMF can effectively improve soil physicochemical properties, increase soil K+ content and reduce soil Na+ content, promoting the uptake and utilization of various mineral nutrients and water required for plant growth and development, thereby enhancing plant salt stress resistance[62,63]. In this experiment, with increasing saline-alkali concentrations, the K+ content of M9-T337 leaves gradually decreased while Na+ content gradually increased. This may be due to ionic competition between Na+ and K+, affecting normal K+ uptake. Meanwhile, saline-alkali stress may impair the selective uptake mechanism of the root system, reducing K+ uptake. This is similar to the findings of the study on citrus by Zhou et al.[64]. After inoculation with AMF treatments, all treatments showed varying degrees of decrease in Na+ content and increase in K+ content. This may be because AMF promoted root growth, improved nutrient uptake, increased water use efficiency, and consequently enhancing saline-alkali tolerance of rootstock M9-T337. This aligns with the findings of the study by Guo et al. on cotton[65].
In conclusion, this study helps to reveal how AMF enhances saline-alkali tolerance in plants at the physiological level, including improving ion homeostasis, enhancing photosynthetic parameters, regulating endogenous hormone homeostasis, and optimizing tissue and stomatal structures. Through these mechanisms, AMF was able to promote the growth and development of apple rootstock M9-T337 under unfavorable environmental conditions and mitigate the negative effects of saline-alkali stress. In addition, this study is instructive for the development and application of biotechnological strategies to improve saline-alkali tolerance in apple and other crops, enhance the sustainability of agricultural production, and expand crop cultivation in salinized areas. Therefore, a comprehensive understanding of the interactions between AMF and apple rootstock M9-T337 holds significant potential for improving apple yield and quality, as well as facilitating adaptation to salinized soil environments.
-
Saline-alkali stress significantly inhibited the growth of apple rootstock M9-T337. Inoculation with AMF can increase the fluorescence parameter values of M9-T337 leaves under saline-alkali stress and maintain photosynthesis of the plant. In addition, it can change various morphological indexes of leaves, increase the hormone content in the plant, and regulate the ionic balance. On the other hand, it can also improve the tissue structure and stomatal morphological structure of leaves, which can alleviate the damage caused by saline-alkali stress to the plant. The comprehensive analysis concluded that AMF could effectively alleviate the inhibitory effect of saline-alkali stress on the growth of rootstock M9-T337. The best effect in alleviating the saline-alkali stress tolerance of rootstock M9-T337 was achieved under M100 treatment. The comprehensive analysis concludes that AMF could effectively alleviate the inhibitory effects of saline-alkali stress on the growth of rootstock M9-T337. The best effect in alleviating the saline-alkali stress tolerance of rootstock M9-T337 was achieved under the M100 treatment.
This work was supported by the Special Fund for National Natural Science Foundation of China (Project Number 32160696), the Gansu Academy of Agricultural Sciences Biological Breeding Special Project (2023GAAS11), and the Student Innovation and Entrepreneurship Training Program of Gansu Province (S202310733049).
-
The authors confirm contribution to the paper as follows: study conception and design, writing original draft: Sun Y; data curation: Sun Y, Han C, Zhai J, Liu B; investigation: Han C; Software: Zhai J; funding acquisition: Sun Y, Xian X, Chen P, Wang Y; writing – review editing: Sun Y, Xian X, Wang Y; supervision: Xian X. All authors reviewed the results and approved the final version of the manuscript.
-
The datasets generated during and/or analyzed during the current study are available from the corresponding author on reasonable request.
-
The authors declare that they have no conflict of interest.
- Copyright: © 2025 by the author(s). Published by Maximum Academic Press, Fayetteville, GA. This article is an open access article distributed under Creative Commons Attribution License (CC BY 4.0), visit https://creativecommons.org/licenses/by/4.0/.
-
About this article
Cite this article
Sun Y, Xian X, Han C, Chen P, Zhai J, et al. 2025. Arbuscular mycorrhizal fungi enhance the saline-alkali tolerance of apple rootstock M9-T337 by regulating chlorophyll fluorescence parameters and hormone balance. Fruit Research 5: e014 doi: 10.48130/frures-0025-0005
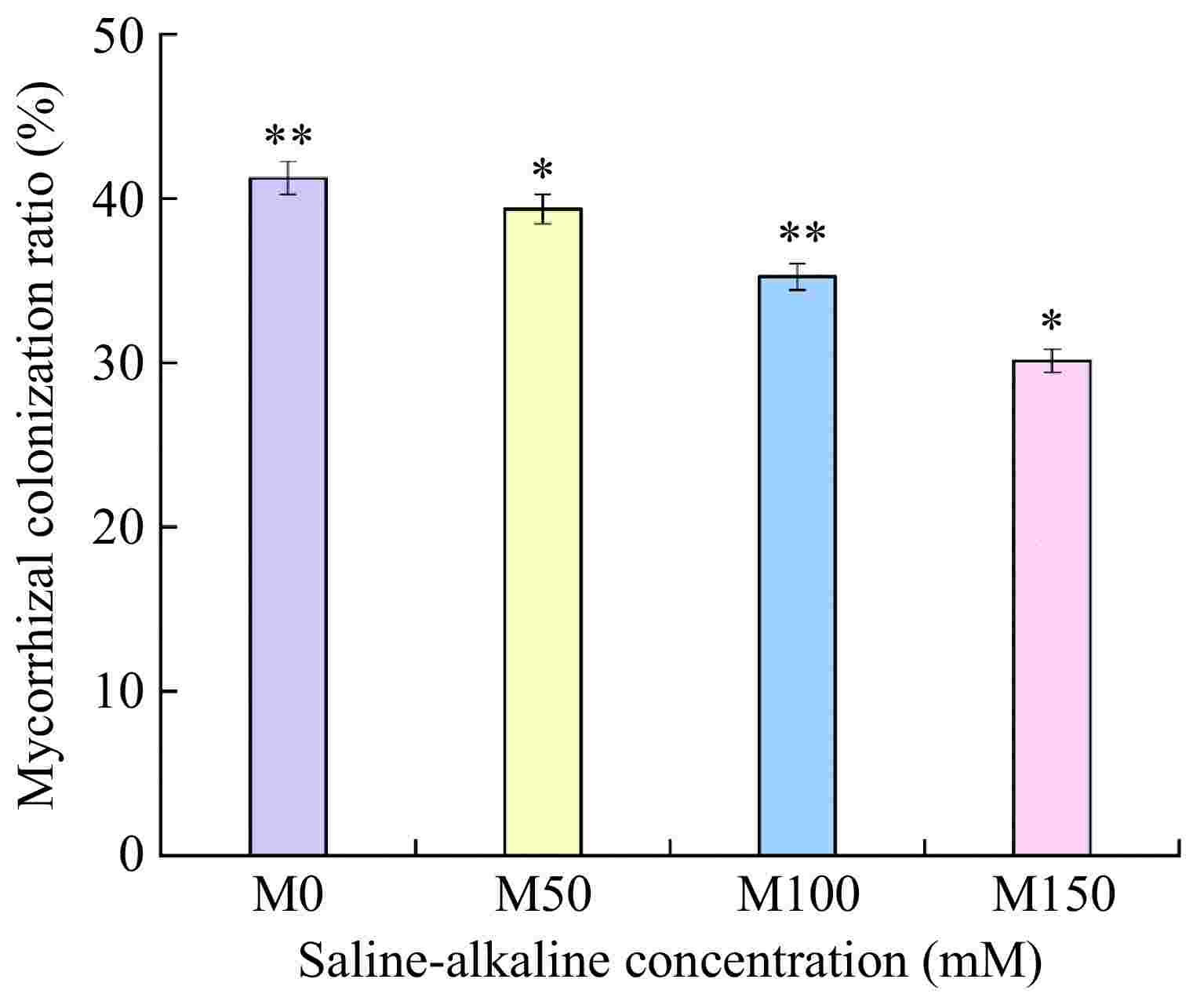