-
Anthocyanins, a type of flavonoid compound that normally exist as glycosides, have been discovered in various plants. Anthocyanins play crucial roles in the physiological and biochemical functions of many horticultural plants, including ornamental plants, fruits, and vegetables[1,2]. They determine the color of fruits, leaves, and flowers, which affects the horticultural traits and benefits to attract pollinators and seed dispersal. Furthermore, anthocyanins serve functions for responding to stress exhibiting antioxidant properties in plants, and providing health benefits for humans[1]. Therefore, they hold significant potential for many applications. Currently, more than 600 varieties of anthocyanins have been identified in plants. The primary types include cyanidin, pelargonidin, and delphinidin, which can give rise to derivatives such as petunidin, malvidin, and peonidin. The coloration of plant tissues is determined by the types and concentrations of these anthocyanin glycosides.
In general, there are three main branches in the biosynthetic pathway of anthocyanin glycosides (Fig. 1), which involve a series of key structural genes encoding enzymes, such as chalcone synthase (CHS), chalcone isomerase (CHI), and flavanone 3-hydroxylase (F3H)[3,4]. Additionally, the biosynthesis of anthocyanins in plants is regulated by transcription factors (TFs) at the transcriptional level. Studies have shown that TFs such as MYB, bHLH, and WD40 can activate the expression of structural genes in the anthocyanin biosynthetic pathway in plants[3]. Furthermore, some MYB TFs that inhibit the expression of structural genes have also been identified[4,5]. More and more new TFs belonging to other families have also been reported recently. However, the differential roles of TFs in the transcriptional regulation of structural genes in horticultural plants are not yet fully understood. This article provides an overview of the functions and biosynthetic pathways of anthocyanins, as well as the regulatory effects of TFs on related structural genes in different horticultural plants. This information offers theoretical guidance for improving horticultural traits, such as flower color, fruit quality, and vegetable characteristics.
-
Anthocyanins, as natural water-soluble pigments and important secondary metabolites, play crucial roles in horticultural plants. They determine the color of flowers, leaves, tubers, rhizomes, and fruits, and offer high nutritional value and health benefits in many crops (Fig. 2)[2]. The flower color of ornamental plants is influenced by the types and concentrations of anthocyanin glycosides. For example, there are three major anthocyanins, including cyanidin, pelargonidin, and peonidin derivatives in the Orchidaceae family. Anthocyanin color can vary under different pH conditions (Table 1), exhibiting red (acidic), purple (neutral), and blue (alkaline) hues[6]. This color variation attracts pollinators such as insects and enhances the ornamental value of horticultural plants[2]. Anthocyanin accumulation can also enhance plant resistance to low temperatures, and cold stress leads to the increase of anthocyanin content.
Table 1. Category, chemical structure, and characteristics of plant anthocyanins[7].
Anthocyanidin Short name R1 R2 R3 Λmax
(nm)pH Color Delphinidin Dp OH OH OH 546 < 3 Blue 3–11 Purple > 11 Red Pelargonidin Pg H OH H 520 Red Cyanidin Cy OH OH H 535 < 3 Red 3–11 Purple > 11 Blue Petunidin Pt OCH3 OH OH 543 < 3 Red 3–5 Pink 5–8 Purple > 8 Blue Malvidin Mv OCH3 OH OCH3 542 Acidic–neutral Red Alkaline Blue Peonidin Pn OCH3 OH H 532 / Deep
red-purpleIn Solanaceous vegetables, purple cultivars such as purple tomatoes, peppers, and eggplants, rich in anthocyanins, exhibit stronger growth, fruit quality, cold tolerance, stress resistance, and disease resistance compared to common varieties. Accumulation of anthocyanins and flavonoids can also enhance the tolerance of vegetable crops to drought, low temperatures, and osmotic stress[8]. For instance, a TF BcMYB44 improved drought resistance of Non-Heading Chinese Cabbage (Brassica campestris) by regulating anthocyanin accumulation, stomatal movement, and homeostasis of reactive oxygen species[8].
In fruits, the color determined by anthocyanins reflects maturity and quality, and anthocyanins can enhance the antioxidant properties of cells and tissues, which benefit human health. Accumulation of anthocyanins in fruit trees also could be induced by low temperatures, drought, and other stresses. For example, drought treatment induced anthocyanin biosynthesis in apple (Malus domestica) seedlings by up-regulating the expression of MdERF38, which encodes a TF interacting with another TF MdMYB1[9]. Low temperatures and light treatments promoted anthocyanin accumulation in grape skin, while the anthocyanin content decreased significantly under opposite conditions. Thus, light is an important factor in regulating anthocyanin biosynthesis in horticultural crops, and supplementary lighting techniques can be employed to manipulate fruit color.
-
Anthocyanins further undergo modifications, including glycosylation, methylation, and acylation, leading to the formation of structurally stable and color-rich anthocyanin glycosides. The anthocyanin biosynthetic pathway (ABP) is a branch of the flavonoid biosynthetic pathway, utilizing phenylalanine as precursor to synthesize anthocyanin glycosides in the cytoplasm and endoplasmic reticulum. Subsequently, they are transported to the vacuole for storage. The structural genes, which encode enzymes involved in anthocyanin biosynthesis, include CHS, CHI, F3H, flavonoid 3'-hydroxylase (F3'H), flavonoid 3',5'-hydroxylase (F3'5'H), dihydroflavonol 4-reductase (DFR), anthocyanidin synthase (ANS), and UDP-glucose flavonoid 3-O-glucosyltransferase (UFGT), and other genes[4]. Anthocyanin biosynthesis in higher plants can be divided into three stages (Fig. 2). In the first stage, using phenylalanine as a precursor, phenylalanine ammonia lyase (PAL), cinnamate 4-hydroxylase (C4H), and 4-coumaroyl-CoA ligase (4CL) catalyze the synthesis of 4-coumaroyl-CoA. In the second stage, 4-coumaroyl-CoA is converted to dihydrokaempferol (DHK) through the catalysis of CHS, CHI, and F3H. Dihydrokaempferol is then converted to dihydroquercetin (DHQ) and dihydromyricetin (DHM) under the action of F3'H and F3'5'H. In the third stage, DHK, DHQ, and DHM are further converted to pelargonidin, cyanidin, and delphinidin, respectively, under the catalysis of DFR and ANS. Subsequently, UFGT catalyzes the formation of pelargonidin-3-glucoside, cyanidin-3-glucoside, and delphinidin-3-glucoside, which are main anthocyanin glycosides. Furthermore, pelargonidin-3-glucoside can be converted to petunidin-3-glucoside and malvidin-3-glucoside, and cyanidin-3-glucoside can be converted to peonidin-3-glucoside, respectively, through the action of O-methyltransferase (OMT). The expression levels of these structural genes can affect the biosynthesis and accumulation of anthocyanin glycosides in plants and are regulated by various TFs.
Structural genes in anthocyanin biosynthesis
CHS and CHI
-
CHS and CHI are key enzymes in the upstream section of the anthocyanin biosynthetic pathway. CHS catalyzes the synthesis of chalcones, which is the initial enzyme reaction in the pathway and provides the fundamental carbon skeleton for the formation of flavonoid compounds. CHI is a crucial enzyme that converts tetrahydroxychalcone to colorless flavanones. The first CHI gene was identified from pea (Pisum sativum) in 1987. The expression levels of CHS and CHI significantly affect the concentrations of total flavonoids in plants. To date, CHS and CHI genes have been identified in many horticultural plants. As reported, the anthocyanin content in plants is also positively correlated with the expression levels of CHS and CHI, and inhibiting the expression of CHS and CHI hinders anthocyanin biosynthesis. For example, over-expression of FhCHS1 from Freesia hybrida in petunia (Petunia hybrida) induced anthocyanin accumulation and the change of flower color from white to pink in transgenic petunia. The expression level of CHS in citrus is significantly positively correlated with flavonoid content, and CitCHS1 can promote flavonoid accumulation in leaves. In mature mulberry (Morus sp.) fruits, the expression levels of MmCHI1 and MmCHI2 are positively correlated with anthocyanin content; and virus induced gene silencing (VIGS) targeting the two CHI genes showed that significant down-regulation of MmCHI2 led to a significant reduction in anthocyanin content (approximately 50%)[10].
2-ODD gene family
-
F3H, ANS, and FLS (flavonol synthase) belong to the 2-Oxoglutarate Dependent Dioxygenase (2-ODD) family, which is a type of non-heme iron-containing enzymes widely in nature. Based on characteristics of amino acid structures, 2-ODDs can be divided into three major classes: DOXA, DOXB, and DOXC. All 2-ODDs related to flavonoid biosynthesis belong to the DOXC sub-branch 28 (F3H) or 47 (FLS and ANS). F3H converts chalcones to dihydroflavonols, which are important precursors for anthocyanin biosynthesis. Down-regulation of F3H in strawberry leads to a significant decrease in the content of dihydroflavonols and anthocyanins. ANS, a key enzyme in the terminal steps of anthocyanin biosynthesis, catalyzes the formation of colored anthocyanins from colorless proanthocyanidins. In raspberry, a 5-bp insertion in the ANS gene leads to gene inactivation and a reduction in anthocyanin content. In magnolia flowers, the transcription level of MsANS is 26 times higher in red petals compared to white petals. FLS, a key enzyme for the biosynthesis of flavonols, catalyzes the formation of flavonols by introducing a double bond between C-2 and C-3 of dihydroflavonols. Because flavonol biosynthesis is another branch of the flavonoid biosynthetic pathway and competes for the substrates with ANS, changes in FLS expression levels may affect the concentrations of anthocyanins. For example, over-expression of FLS genes from Petunia hybrida, Rosa rugosa, Prunus persica, and Chrysanthemum morifolium induced increased flavonols, decreased anthocyanins, and paler flower color in petals of transgenic tobacco[11]. Studies have shown that high amino acid similarity between different enzymes may result in functional overlap. For example, FLSs from Ginkgo biloba and rice can use both flavonols and dihydroflavonols as substrates, while ANSs from Arabidopsis thaliana and apple can catalyze the formation of flavonol glycosides[12].
F3′H and F3′5′H
-
F3′H and F3′5′H belong to the cytochrome P450 gene superfamily and are classified into the CYP75B and CYP75A subfamilies, respectively. They catalyze the hydroxylation of dihydrokaempferol to form dihydroquercetin and dihydromyricetin, which can further be converted to cyanidin and delphinidin by downstream enzymes. Therefore, F3′H and F3′5′H are key genes determining flower color. In dicotyledonous plants, F3′5′H derived from F3′H through at least four evolutionary events, representing new catalytic functions of enzymes. F3′H is widely distributed in higher plants, while the distribution of F3′5′H is more scattered. The F3′H and F3′5′H genes have been cloned and functionally analyzed in various plants. In poinsettia, knockout of the F3′H gene using CRISPR/Cas9 technology resulted in a color change from red (cyanidin) to orange (pelargonidin) in bracts, with a significant decrease in cyanidin content and a relative increase in pelargonidin content compared to the wild type[12]. In grapes, the F3′5′H gene is expressed in different tissues of the plant containing flavonoids, especially in the mature grape skins with the highest concentrations of anthocyanins[13]. In Delphinium plants, F3′5′H is highly active, resulting in blue or purple flowers containing high content of delphinidin-derived pigments, whereas F3′H is absent, lead to lack of red or pink flowers.
DFR and UFGT
-
DFR converts dihydroflavonols, including dihydrokaempferol, dihydroquercetin, and dihydromyricetin, into colorless leucoanthocyanidins, and plays a crucial role in the coloration of plant tissues. DFR was initially identified and cloned from maize. DFR competes for substrates with F3′H and F3′5′H. High expression of DFR and F3'H can inhibit the expression of F3'5'H, leading to a preference for accumulation of pelargonidins in plants. UFGT is located downstream of the anthocyanin biosynthetic pathway. UFGT catalyzes the formation of anthocyanin-3-glucosides by transferring glucose moieties from UDP-glucose to the C3 hydroxyl of cyanidin, pelargonidin, and delphinidin. Expression of UFGT in horticultural plants is associated with the coloration of plant organs such as fruits and flowers. During the flowering process of peach, the total anthocyanin content initially increases at the full flowering stage and then decreases at the late flowering stage, associating with the same expression pattern of the UFGT gene. In the peel of lychee fruit, there is a strong positive correlation between UFGT expression and the content of anthocyanins and flavonoids.
-
Currently, the biosynthesis of anthocyanins in most horticultural plants is directly regulated by three classes of TFs or their complex, known as MYBs, bHLHs, and WD40 proteins. Among these three TFs, MYBs normally play predominant roles. Additionally, other TFs such as WRKY, ZIP proteins, MADS-box, and NAC proteins also participate in the regulation of anthocyanin biosynthesis, by interacting with the MYB-bHLH-WD40 (MBW) complex or directly regulating members of MBW at transcriptional level (Table 2)[14].
Table 2. Other TFs involved in the regulation of anthocyanin biosynthesis.
TFs family Plant species Representative TFs Targets Ref. NAC Malus domestica MdNAC42 MYB [15] NAC Prunus persica BL/PpNAC1 MYB [16] NAC Arabidopsis thaliana ANAC032 DFR, ANS/LDOX,
and TT8[17] MADS-box Vaccinium myrtillus VmTDR4 MYB [18] bZIP Solanum lycopersicum SlHY5 CHS, DFR [19] bZIP Malus domestica MdHY5 MYB [19] WRKY Petunia hybrida PhPH3 MYB complex [20] SPL Salvia miltiorrhiza SmSPL7/SPL9 4CL [21] SPL Arabidopsis thaliana AtSPL9 MBW complex [21] Ornamental plants
-
In the anthocyanin biosynthesis pathway of ornamental plants, transcription factors such as MYB, bHLH, and WDR can individually or cooperatively regulate the transcription levels of structural genes and affect the formation of flower color. For example, the flower color of petunia is determined by four MYB proteins, namely PhAN2, PhAN4, PhPHZ, and PhDPL. Among them, PhAN2 determines the red color of the corolla, while PhAN4 induces anthocyanin accumulation in anthers and corolla tubes by interacting with a bHLH transcription factor PhAN1. Although PhDPL and PhPHZ activate the transcription levels of the same structural genes, PhDPL determines pigment accumulation and vein patterns in the corolla tube, and PhPHZ determines the red coloration of flower buds. In Japanese morning glory, MYB1, a homolog of PhAN2, activates the expression of anthocyanin-related genes in the branches and flower tubes by interacting with WDR1 and bHLH2.
There are two anthocyanin-regulating MYBs (MgPELAN and MgNEGAN) in monkey flower (Mimulus guttatus)[22], three anthocyanin-regulating MYBs (AmVENOSA, AmROSEA1, and AmROSEA2) in snapdragon (Antirrhinum majus). In lilies and orchids, anthocyanin biosynthesis also can be activated by MYBs, of which different members have phylogenetic relationships, with slight functional differences[23]. Aside from the flower-based ornamental plants mentioned above, the color change in the spathes of Anthurium andraeanum is also regulated by AaMYB2, which primarily activates the transcription levels of AaF3H and AaANS.
In ornamental plants, MYB repressors that inhibit anthocyanin biosynthesis have also been identified. There are two types of MYB repressors, R2R3-MYB proteins, and R3-MYB proteins. Normally, R2R3-MYB proteins directly repress the expression of anthocyanin-related genes by binding to their promoters. For example, PhMYB27 targets both structural genes and the bHLH PhAN1 in petunia. In chrysanthemum, CmMYB21 and CmMYB3-like repress the expression of structural genes directly, resulting in decreased anthocyanins in color-fading petals[24]. CmMYB012 inhibits anthocyanin biosynthesis in response to high temperatures in chrysanthemum. CmMYB4 which can be induced by a low R/FR light ratio, represses anthocyanin activator CmbHLH2, leading to the reduction of anthocyanin accumulation in chrysanthemum petals. However, R3-MYB proteins can not bind to promoters, and act as repressors by interacting with bHLHs in MBW complexes. Normally, these R3-MYB proteins regulates anthocyanin biosynthesis indirectly, such as PhMYBx in petunia, CmMYB#7 in chrysanthemum. To date, only a few anthocyanin-related MYB regulatory factors have been identified in some important ornamental plants, and there is a scarcity of MYB inhibitors. Therefore, the identification and functional analysis of MYB TFs in non-model ornamental plants are particularly important.
Fruit tree crops
-
So far, most transcription factors such as MYB, bHLH, and WDR play a promoting role in the biosynthesis of anthocyanins in fruits, while a few regulatory factors can inhibit anthocyanin biosynthesis. In Chinese bayberry (Myrica rubra), the transcription levels of MrMYB1 positively correlate to the anthocyanin content in mature fruits, thereby determining different fruit colors such as white, red, and deep purple-red. Phylogenetic analysis has revealed that the sequences of kiwifruit AcMYB110a, grape VvMYBA1, VvMYBA2, and bayberryi MrMYB1 show high homologous and all strongly influence the anthocyanin accumulation in fruits. In apple, MdMYB1, MdMYB10, and MdMYBA up-regulate the expression of structural genes to increase the anthocyanin content in the fruit skin[25], while MYB110a regulates the formation of red color in apple flesh. In cherry (Prunus avium), PavMYB activates the promoters of late-stage synthesis genes involved in the anthocyanin pathway through interactions with bHLH and WD40[26]. Moreover, the regulatory role of MYB in anthocyanin biosynthesis is tissue-specific. In fruits, MYBs within the same species exhibit higher homology compared to MYBs between different species, and MYBs that inhibit anthocyanin biosynthesis in different fruits are also highly related. For example, VvMYBC2L1 and VvMYBC2-L3 in grapes belong to the same branch with MYB inhibitors of anthocyanin in peach and strawberry, which can inhibit the accumulation of proanthocyanins by down-regulating structural genes[27]. In addition to the WDR, bHLH, and MYB transcription factor families, other transcription factors can also influence anthocyanin biosynthesis in fruit trees, such as BBX16, BBX18, and BBX21 in pear (Pyrus pyrifolia)[28], MdWRKY11, MdNAC42, MdERF38, MdIAA26, and MdHB1 in apple[14], PpNAC1 in peach (Prunus persica)[16].
Vegetable crops
-
Anthocyanins accumulate in the flowers, leaves, roots, stems, and fruits of many vegetable crops, such as cabbage, cauliflower, and purple kale in the Brassica genus. In cabbage, BoMYB1, BoMYB2, and BoMYB3 are involved in anthocyanin biosynthesis, and BoMYB2 possibly up-regulates the transcription levels of structural genes in red cabbage through interaction with a bHLH. In the same branch of the phylogenetic tree, RsMYB1 also induces the expression of structural genes through interaction with a bHLH to regulate anthocyanin biosynthesis in radish (Raphanus sativus)[29]. MYBs also play key roles in anthocyanin accumulation in Solanaceae crops. In tomato, two MYBs (SlANT1 and SlANT2) are involved in the biosynthesis of lycopene and are mainly responsible for regulating purple coloration in vegetative tissues and fruits. However, the anthocyanins produced by SlANT2 are only visible under high light or low-temperature conditions. In eggplant (Solanum melongena), SmMYB1, the ortholog of tomato SlANT1, also up-regulates the expression of most structural genes to regulate purple coloration in fruits, and overexpression of SmMYB1 leads to higher anthocyanin content in transgenic eggplants[30]. In some vegetable crops, the regulation of MYB on anthocyanin biosynthesis may be induced by external environmental factors. For example, in mustard (Brassica juncea), the expression levels of four MYBs in seedlings grown under light are significantly higher than those grown in the dark. Under high-temperature conditions, the potato StMYB44s does not interact with StbHLH and directly inhibits the activity of the DFR promoter, leading to a decrease in anthocyanin content in potato tubers[31].
-
Some plant hormones are involved in anthocyanin biosynthesis in horticultural plants. For instance, in ripening apple fruit, ethylene regulates anthocyanin biosynthesis by inducing the antagonistic activities of the R2R3-MYB repressor like MdMYB17 and activators like MdMYB1 and MdEIL1[32]. Ethylene-induced MdEIL1 up-regulates MdERF1B, which then directly up-regulates anthocyanin activators MdMYB1/9/11[33]. The crosstalk between auxin and ethylene signals in regulating anthocyanin biosynthesis was also revealed[34]. Jasmonic acid (JA) degrades MdJAZ5/10, which interacts with MdERF1B and inhibits MdMYB1/9/11 promoter activation[33]. Therefore, ethylene, and JA cooperate to modulate anthocyanin production in apple[33]. Besides, ABA induced the expression of MdNAC1, which encodes an NAC transcription factor interacting with a bZIP-type transcription factor MdbZIP23 to promote anthocyanin biosynthesis in red-fleshed apples[35]. MdbHLH162 can integrate gibberellin (GA) and JA signals to negatively regulate anthocyanin biosynthesis[36]. And MdNAC72 also can integrate JA and GA signaling to regulate anthocyanin biosynthesis in apple[37]. The role of SL (strigolactone)-GA crosstalk in regulating anthocyanin biosynthesis in apple has also been revealed[38].
Anthocyanin biosynthesis in horticultural plants is also regulated by environmental signals. Normally, anthocyanins in plants could be induced by low temperatures, and high UV lights, and be reduced by high temperatures[39]. Low environmental temperatures promote anthocyanin accumulation and fruit coloration of apple fruits, by inducing MdbHLH3 which activates the expression of MdDFR, MdUFGT, and the regulatory gene MdMYB1[40]. Under low-temperature conditions, the small ubiquitin-like modifier E3 ligase MdSIZ1 promotes anthocyanin accumulation by sumoylating MdMYB1 in apple[41]. However, in strawberry fruit, low temperature activates FvMAPK3-induced phosphorylation of FvMYB10 and degradation of CHS1 and inhibits anthocyanin accumulation[42]. High temperatures inhibit anthocyanin biosynthesis and expression of related genes in several diverse plant species, such as apple[43], and 'Kyoho' grapevines[44]. In potato, heat stress reduced the expression of the activators R2R3-MYB StAN1 and StbHLH1, but induced the expression of the repressor StMYB44[31]. The light environment is another critical factor affecting anthocyanin biosynthesis. Blue light and 24-h irradiation together promoted anthocyanin biosynthesis in pepper leaves, by inducing the expression of key genes in the anthocyanin synthesis pathway[45]. In chrysanthemum 'Purple Reagan', shading treatment largely reduced the anthocyanin content in chrysanthemum capitulum, suggesting that anthocyanin biosynthesis depends on light[46]. A low red-to-far red (R/FR) light ratio reduces anthocyanin accumulation, whereas a high R/FR light ratio promotes anthocyanin biosynthesis in chrysanthemum. A low R/FR light ratio induced the expression of CmMYB4, which suppressed the anthocyanin activator complex CmMYB6-CmbHLH2, whereas a high R/FR light ratio up-regulated CmbHLH16 which impeded the formation of the CmMYB4-CmTPL complex and released the suppression of CmbHLH2[47].
-
Anthocyanins are garnering widespread attention because they play important roles in coloration and many other physiological functions in horticultural plants, and benefit human health with antioxidant properties. The basic structure of anthocyanins and the mechanism of biosynthesis have been explored and improved for many years. However, the biosynthesis pathway of anthocyanin glycosides is complex and regulated by different transcription factors, as well as environmental factors such as temperature, light, and drought. Therefore, further research is needed to deepen our understanding of the regulatory mechanisms. In many horticultural plants, structural genes such as CHS, CHI, and F3H are composed of small gene families, but research on these families is relatively limited. Future studies should focus on conducting in-depth investigations into structural genes involved in the anthocyanin biosynthesis pathway in a wider range of horticultural plants. Regarding transcriptional regulation, current research is mostly about the regulatory role of R2R3-MYB transcription factors, especially transcription activators. However, there is relatively limited information on transcription factors that negatively regulate anthocyanin biosynthesis. Subsequent studies should further explore other transcription factors related to anthocyanin biosynthesis in horticultural plants and elucidate the regulatory mechanisms between transcription factors and structural genes, as well as among transcription factors and environmental factors. This will provide a theoretical basis for improving the coloration of plant tissues such as flowers, leaves, and fruits. With the continuous development of technological approaches such as genomics, transcriptomics, metabolomics, conjoint analysis, and bioinformatics analysis, more new genes related to the biosynthesis and regulation of anthocyanins could be discovered. Additionally, the application of gene editing and genetic engineering technologies can be employed to manipulate genes involved in the anthocyanin biosynthesis pathway in a wider range of horticultural plants. These efforts will lay the foundation for regulating the coloration of flowers and leaves in ornamental plants, and for breeding new varieties of fruits and vegetables with high anthocyanin content.
This study was financially supported grants from introduction of talent projects of Anhui Science and Technology University (JZYJ202201), 'Pioneer' and 'Leading Goose' R&D Program of Zhejiang (2023C02028-1), Natural Science Foundation of Zhejiang Province (LQ22C150008), the Foundation of Key Laboratory of Biology of Ornamental Plants in East China, National Forestry and Grassland Administration (KFE202403), Anhui Provincial University Research Project (2024AH050326), and Anhui Science and Technology University Student Innovation and Entrepreneurship Training Program (202310879071, S202310879153, 202410879058).
-
The authors confirm contribution to the paper as follows: draft manuscript preparation: Zhao K, Wang Yuting, Zhang Q, Wei Q; manuscript editing & review: Zhao K, Wang Yunzhu. All authors have read and agreed to the published version of the manuscript.
-
All data included in this study are available upon request from the corresponding author.
-
The authors declare that they have no conflict of interest.
-
Received 13 August 2024; Accepted 30 December 2024; Published online 24 March 2025
- Copyright: © 2025 by the author(s). Published by Maximum Academic Press on behalf of Hainan University. This article is an open access article distributed under Creative Commons Attribution License (CC BY 4.0), visit https://creativecommons.org/licenses/by/4.0/.
-
About this article
Cite this article
Zhao K, Zhang Q, Wang Y, Wei Q, Wang Y. 2025. Advances in the biosynthesis and regulatory mechanisms of anthocyanins in horticultural plants: a comprehensive review. Tropical Plants 4: e010 doi: 10.48130/tp-0025-0002
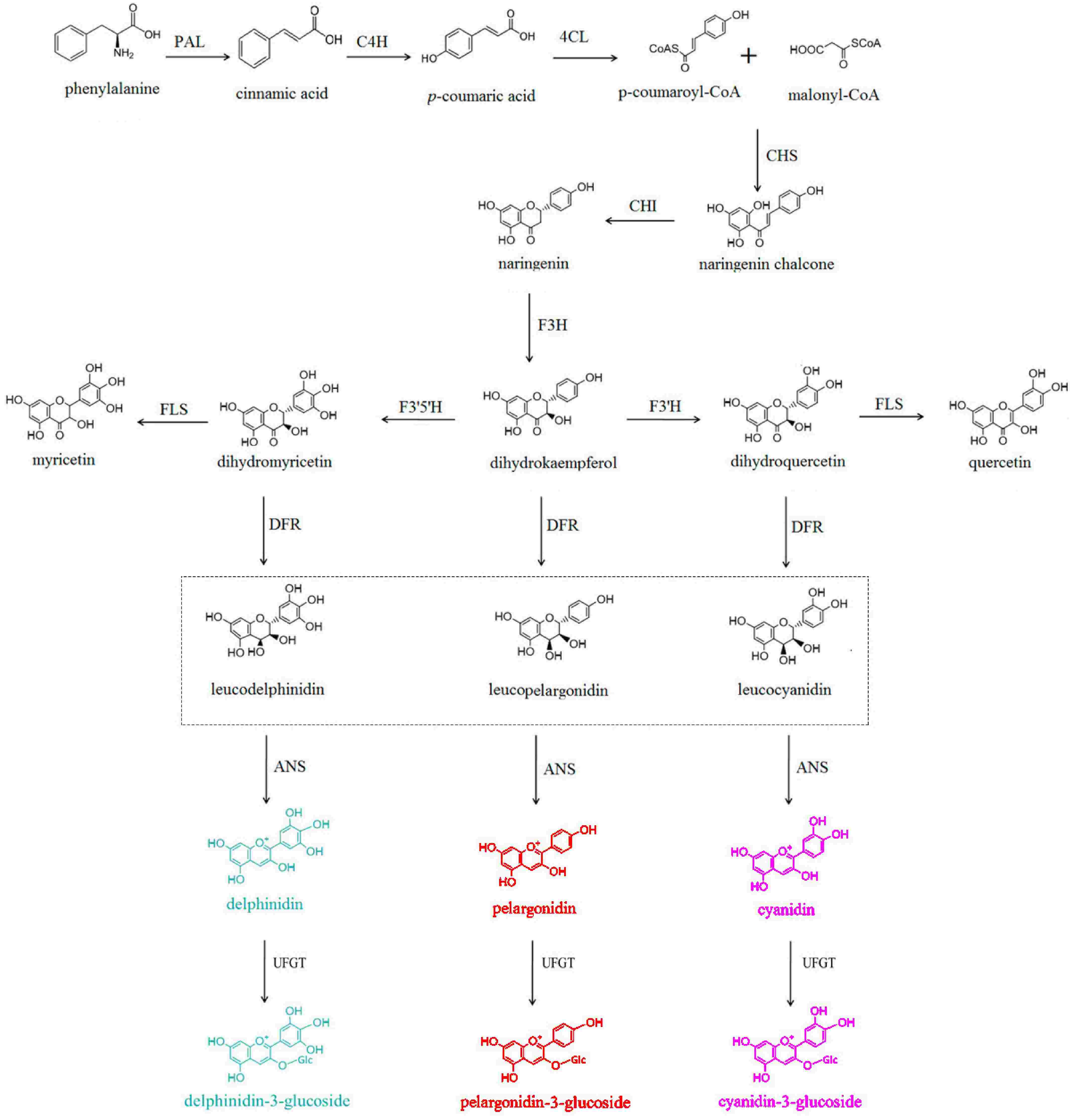