-
Flavonoids, as important plant secondary metabolites, exhibit a broad spectrum of biological effects, such as antioxidative, anti-inflammatory, and anti-atherosclerotic activities. Their exceptionally low toxicity has attracted considerable interest for their potential in health food and pharmaceutical development[1−3]. The biological function of flavonoids is closely linked to their chemical structures. Unmodified flavonoids possess limited bioavailability, which greatly hinders their clinical application and chemopreventive potential[4,5]. However, O-methylation has been shown to effectively enhance the bioavailability of flavonoids[6]. For example, EGCG3′′Me((−)-epigallocatechin-3-O-(3-O-methyl)-gallate), an O-methylated EGCG from tea, has better antiallergy[7] and antihypertensive[8] effects than a tea without O-methylated EGCGs. Compared with unmethylated baicalein, the poly-methoxylated 4'-deoxyflavones, such as skullcapflavone I and tenaxin I, exhibited more potent apoptosis-inducing abilities without toxic side effects on healthy cells[9]. This important modification reaction often occurs at specific sites on the B ring (3'-OH and 4'-OH) and A ring (6-OH, 7-OH, and 8-OH) of flavonoids[10]. O-methylation not only diversifies the species of flavonoids but also increases their hydrophobicity, improves lipid, and protein affinity, enhances membrane transport capacity, promotes absorption, and ultimately enhances stability during metabolism and bioavailability[11,12].
The methylation process, which is catalyzed by S-adenosyl-L-methionine (SAM)-dependent O-methyltransferase (OMT), plays a crucial role in the biosynthesis of O-methylated flavonoids[9,13]. Plant OMTs exhibit a wide range of biological functions and substrate specificity, and can be divided into two main subgroups according to their molecular masses and enzymatic characteristics[14]: the caffeoyl coenzyme A OMT (CCoAOMT) subfamily, which is Mg2+-dependent and has a molecular weight ranging from 26 to 30 kDa, is distinct from the caffeic acid OMT (COMT) subfamily, which has a higher molecular weight of 38 to 42 kDa. Most OMTs related to flavonoids are members of the COMT subfamily[13,15−18]. Furthermore, the CCoAOMT subfamily can be subdivided into two groups: one is the CCoAOMT-like (PFOMT) subgroup, which participates in the methylation of flavonoids, and the other consists of true CCoAOMTs, as identified by Ibdah et al.[19], which are responsible for catalyzing the methylation of caffeoyl-CoA to biosynthesize lignin in vivo[20]. Therefore, phylogenetic analysis offers valuable insights into the putative functions of selected enzymes.
Musella lasiocarpa, a perennial large clustered herb of the Musaceae family in the genus Musella, is primarily found in southwestern China[21]. Traditionally utilized for medicinal, food, and fodder purposes[22], M. lasiocarpa has been shown through phytochemical research to contain flavonoid and phenolic compounds as its main chemical composition responsible for its medicinal effects[23]. Therefore, investigating methylated flavonoid biosynthesis serves as a valuable guide for harnessing the medicinal potential of M. lasiocarpa. We previously reported the genome of M. lasiocarpa and identified three O-methyltransferases (Ml01G0494, Ml04G2958, and Ml08G0855) that can catalyze the methylation of phenylphenatenones[24]. However, the methylation processes related to the biosynthesis of flavonoids in M. lasiocarpa remain unknown. In this study, four O-methyltransferase genes with high expression and complete sequence were selected as candidate genes by using genome and transcriptome association analysis, which were named Ml01G0494, Ml04G2958, Ml05G3914, and Ml08G0855. These four candidate OMTs were expressed and purified in Escherichia coli and tested for their catalytic activity in vitro with several possible substrates. Our findings provide insights into methylated flavonoid biosynthesis in M. lasiocarpa, facilitating functional breeding of M. lasiocarpa with enhanced properties related to methylated flavonoid production, and may promote factory-scale production of O-methylated flavonoid through metabolic engineering.
-
The M. lasiocarpa specimens were gathered from Nanhua County, Yunnan Province, China (118°50′38″ E, 32°3′44″ N) and then relocated to the experimental field at Nanjing Botanical Garden Mem. Sun Yat-Sen, Nanjing, China (101°1′2″ E, 25°9′54″ N). It was identified as M lasiocarpa of the Musaceae family by Yu Chen, a research fellow from the Institute of Botany, Jiangsu Province (China), and the Chinese Academy of Sciences (Beijing, China).
Gene cloning and protein expression
-
The RNAprep Pure Plant Plus Kit (Tiangen Biotech, China) was used to extract total RNA, which was then reverse-transcribed into cDNA using the Evo M-MLV RT for PCR Kit (Accurate Biology, China). The primers used for cloning the target fragments are listed in Supplementary Table S1. The coding DNA sequences of MlOMT candidate genes were inserted into pMAL-c4x (EcoR I / Sal I) to create recombinant plasmids. Sanger sequencing was performed to confirm the identity of the recombinant plasmids before introducing them into E. coli BL21 (DE3) for protein expression. Engineered E. coli strains containing the recombinant plasmids were cultured in 5 mL Luria-Bertani (LB) medium with ampicillin antibiotics at 37 °C for 12 h. Then, 1 mL of the seed broth was transferred to 100 mL LB and incubated at 37 °C for 3 h. Induction of the engineered strains was achieved by adding 0.1 mM IPTG, followed by an additional incubation period of 18 h at 16 °C. The cells were collected by centrifugation at 6,000 rpm for 5 min at 4 °C, and then suspended in 10 mL of binding buffer (pH 7.5, containing 100 mM Tris–HCl, 10 mM MgCl2, 2 mM DTT, and 10% glycerin). The suspension was homogenized on ice for 20 min using a sonicator set to a power of 38%, with intervals of working for 3 s followed by resting for 5 s. After centrifugation at 12000 rpm for 30 min at 4 °C, the cell debris was removed. The supernatant was purified using an ÄKTA protein purification device and the protein was concentrated using Amicon Ultra-15 Ultracel-30K centrifuge filters (Merck Millipore). Protein detection was performed by sodium dodecyl sulfate-polyacrylamide gel electrophoresis (SDS-PAGE) using One-Step PAGE Gel Fast Preparation Kit (12%, Vazyme, China).
HPLC-Q TOF-MS/MS analysis of enzymatic reaction products
-
Assays of methylation activities were conducted in a reaction mixture containing 20 μL purified protein, 0.1 mM substrate, 5 mM SAM, and 25 mM sodium vitamin C in 100 mM Tris-HCl buffer (pH 7.5, 100 μL). The pMAL-c4x empty vector served as the negative control. After being incubated at 37 °C for 12 h, the reaction was terminated by adding an equal volume of methanol and subjecting it to ultrasound for 30 min. The resulting suspension was then used for product identification via high performance liquid chromatography-quadrupole time-of-flight mass spectrometry (HPLC-Q TOF-MS/MS, Agilent, USA) after centrifugation at 12,000 rpm for 15 min. Chromatography was performed with an Agilent Poroshell C18 column (4.6 mm × 100 mm length, 2.7 μm pore size). The gradient elution parameters were established as follows: phase A consisted of water with 0.1% formic acid, while phase B was composed of methanol. The elution process involved the following steps: 2 min at 10% B, 7 min at 60% B, 9 min at 60% B, 12 min at 100% B, 13 min at 100% B, 14 min at 10% B, and 16 min at 10% B. The flow rate was maintained at a constant level of 0.3 mL/min and an injection volume of 5 μL was used. Mass spectra were obtained in positive ion mode with specific acquisition parameters: a capillary voltage of 4 KV; drying gas flow rate set to 10 L/min; drying gas temperature held steady at 350 °C; fragmentor voltage set to 120 V; and nebulizer pressure maintained at 35 psi. Mass spectra were recorded within the range of 100 to 1,500 m/z, and collision energies varied from 10 to 60 eV. Compounds were identified by comparing molecular ions and fragmentation behaviors with reference standards or compounds identified in the existing literature.
-
Based on the genome and seed transcriptome data (GeneBank: PRJNA1009687) obtained in the early stages of our research, we analyzed the gene expression levels (FPKM) at seed developmental stages S2, S4, and S6. The expression level data of OMT gene family members at these different developmental stages was normalized to a range of 0−1[24,25]. Subsequently, four highly expressed genes Ml01G0494, Ml04G2958, Ml05G3914, and Ml08G0855 with relative expression levels exceeding FPKM > 50 were identified as candidate genes for cloning and subsequent construction onto the prokaryotic expression vector pMAL-c4x.
Phylogenetic analysis of M. lasiocarpa O-methyltransferases
-
The alignment of amino acid sequences showed that the key residues of the four cloned OMTs, including the SAM interaction region and four conserved motifs (labeled I–IV) typical of the SAM-binding site in plant SAM-dependent methyltransferases, were highly conserved[26]. The residues in these motifs that have direct interaction with SAM, including V215 in motif I, D229 in motif II, G248 in motif III, and K263 in motif IV, were observed to be conserved across the four MlOMTs examined, except Ml08G0855 (Fig. 1). Additionally, the residues involved in substrate binding (M128 and V314), as well as the crucial catalytic residue E327, were similarly preserved in both Ml01G0494 and Ml04G2958[27]. Furthermore, it was noted that the SAM-binding motif I in all MlOMTs contained a highly conserved glycine-rich sequence of 'G × G × G'.
Figure 1.
Multiple sequence alignment of MlOMTs and AtCOMT1. Asterisks indicate conserved residues, namely substrate binding sites (M128, V314, I317, M318), SAM binding sites (V215, D229, G248, K263), a critical residue (E327) in AtOMT1. Conserved motifs I–IV are underlined. At, Arabidopsis thaliana.
Conserved domain analysis was performed using NCBI-Conserved Domains, E-value < 0.01. The results showed that all candidate proteins contained SAM-dependent methyltransferase conserved domains and belonged to the methyltransferase superfamily of SAM or AdoMet-MTase. A diagram of conserved domain analysis is shown in Supplementary Fig. S1.
To analyze the evolutionary relationship and possible biological functions of the candidate genes, the amino acid sequences of OMT from other species were selected and compared with the candidate genes, and a phylogenetic tree (Maximum Likelyhood) was constructed by MEGA11 software. Set the parameter bootstrap value to 1000. The phylogenetic tree is shown in Fig. 2. The results showed that Ml01G0494, Ml04G2958, and Ml05G3914 were clustered into a branch with members belonging to COMT subfamily, which may have the function of catalyzing flavonoid methylation. Ml08G0855 is clustered with members of the trure-CCoAOMT isoform of the CCoAOMT subfamily and may be involved in lignin biosynthesis.
Figure 2.
Phylogenetic analysis of MlOMTs and other plant OMT proteins. The GenBank accession numbers of OMT proteins from other plants are listed in Supplementary Table S2.
In vitro enzyme assays of the recombinant Ml01G0494, Ml04G2958, Ml08G0855, and Ml05G3914
-
The protein expressed by the constructed engineered E. coli was detected by SDS-PAGE. The molecular weight of OMT ranges from 23 to 43 kDa, with the addition of a 42 kDa MBP tag resulting in a relative molecular weight for recombinant OMT between 63 and 83 kDa. The electrophoresis results for Ml01G0494, Ml04G2958, Ml08G0855, and Ml05G3914 are depicted in Fig. 3. It is evident that a substantial amount of soluble protein is expressed in the supernatant, exhibiting consistent molecular weights with the target proteins and displaying single purified bands, indicating effective purification.
Figure 3.
SDS-PAGE analysis of recombinant Ml01G0494, Ml08G0855, Ml04G2958, and Ml05G3914 proteins. (a) M: protein molecular marker; Lane 1: pMAL-c4x sediment (MBP ≈ 42 kDa); Lane 2: pMAL-c4x supernatant; Lane 3: Ml01G0494 sediment; Lane 4: Ml01G0494 supernatant; Lane 5: Ml01G0494 purified recombinant protein; Lane 6: Ml08G0855 sediment; Lane 7: Ml08G0855 supernatant; Lane 8: Ml08G0855 purified recombinant protein. (b) M: protein molecular marker; Lane 1: pMAL-c4x sediment; Lane 2: pMAL-c4x supernatant; Lane 3: Ml04G2958 sediment; Lane 4: Ml04G2958 supernatant; Lane 5: Ml04G2958 purified recombinant protein; Lane 6: Ml05G3914 sediment; Lane 7: Ml05G3914 supernatant; Lane 8: Ml05G3914 purified recombinant protein.
We have previously identified MI08G0855, M101G0494, and M104G2958 as catalysts for the O-methylation of phenylphenalenones (PhPNs). However, this discovery does not fully elucidate the biosynthetic pathway of O-methylated flavonoids in M. lasiocarpa. Therefore, we conducted a reanalysis and discovered a novel COMT, Ml05G3914. Then selecting several flavonoids (quercetin, baicalein, myricetin, luteolin naringenin, rutin, apigenin, and kaempferol) and one phenylpropionic acid compound as substrates to investigate the enzyme's substrate selectivity. HPLC-Q TOF-MS/MS analysis was employed to examine both the products generated by the recombinant enzyme and their respective substrates.
It was found that Ml01G0494, Ml04G2958, and Ml05G3914, all three enzymes can catalyze the monomethylation of baicalein and quercetin. HPLC-Q TOF-MS/MS analysis validated that the monomethoxy-baicalein product m/z 285.0848 [M + H]+ and monomethoxy-quercetin product m/z 317.0741 [M + H]+, along with a substrate peak at m/z 271.0910 and 303.0620 [M + H]+ respectively (Fig. 4a, b). Compared to Ml05G3914, enzymes Ml01G0494 and Ml04G2958 exhibited higher catalytic activity towards baicalein and quercetin. Enzymes MI08G0855, Ml01G0494, Ml04G2958, and Ml05G3914 were all capable of catalyzing the formation of monomethoxy-luteolin with a product ion of m/z 301.0724 [M + H]+, as well as monomethoxy-caffeic acid with a product ion of m/z 195.0707 [M + H]+. Similarly, Ml01G0494 and Ml04G2958 demonstrated superior catalytic activity towards caffeic acid and luteolin compared to Ml05G3914 (Fig. 4c, d). Ml05G3914, Ml01G0494, and Ml04G2958 efficiently catalyze the two-step O-methylation of myricetin to dimethoxy-myricetin with high conversion rates, exhibiting negligible formation of monomethoxy intermediates. Conversely, MI08G0855 demonstrates a limited capacity to simultaneously methylate multiple hydroxyl groups on myricetin, as evidenced by the copresence of both monomethoxy and dimethoxy product peaks in HPLC-Q TOF-MS/MS chromatograms (Fig. 4e). Overall, the findings indicated that MlOMTs exhibited broad regioselectivity in catalyzing methylation reactions for quercetin, baicalin, luteolin, caffeic acid, and myricetin. The catalytic reaction diagram is shown in Fig. 5 below. The dashed arrows indicate the possible catalytic sites guessed based on the literature[28]. Subsequent standard products need to be purchased for structural confirmation.
-
Flavonoids and their methylated derivatives represent significant classes of phytochemicals, exhibiting a diverse array of structures and biological properties. These compounds contribute to pigment formation[29] and hormone signaling[30], and they also play important roles in defense against various biotic and abiotic stresses[29,31], including protection against pests and diseases, drought tolerance, frost resistance, and protection against UV-B radiation[32,33]. Due to their multiple functions for human health, including anticancer, antimutagenic, antimicrobial, antiviral, and antioxidant activities[28], they have gained increasing attention in recent years. Methylation plays a pivotal role in deactivating the reactive hydroxyl groups of flavonoids, modifying their solubility and intracellular localization[34,35], and enhancing their lipophilicity[14], thereby impacting their biological activities. For example, Mulati et al. have demonstrated that isorhamnetin (3'-O-methylquercetin) effectively mitigates cognitive dysfunction and neuroinflammation induced by high fat and high sugar consumption[36]. Studies have also demonstrated a direct correlation between the tumor inhibitory activity and the number of methoxy substitutions, with higher levels of substitutions resulting in increased tumor inhibitory activity[37]. The identification and functional characterization of OMTs involved in flavonoid methylation has been performed in many plant species, especially in medicinal plants, including Catharanthus roseus[15], Scutellaria baicalensis[9], Citrus reticulata cv. Chachiensis[38], and Isatis tinctoria[39]. However, it is challenging to harness the health benefits of O-methylated flavonoids in plants due to their relatively low natural content. The large-scale synthesis of flavonoids and their derivatives frequently rely on genetically engineered microorganisms to facilitate efficient production. Extensive research has shown that M. lasiocarpa possesses various health-promoting properties, such as anti-inflammatory, hemostatic, anti-enteritis, anti-constipation, detoxification, and anti-gynecological disease effects[22,40]. Moreover, it serves as a valuable source of nutrients, and its extensive nutritional content and utilization in the traditional food and swine diets of the Yi people were documented in a previous study[41]. To harness the potential of M. lasiocarpa's genetic repository, it is imperative to isolate OMT genes to facilitate the industrial synthesis of O-methylated flavonoids through heterologous expression in engineered microorganisms like E. coli.
In this study, four flavonoid substrates and caffeic acid were selected to investigate the regioselectivity of MlOMTs. Ml05G3914, Ml01G0494, and Ml04G2958 can directly catalyze the two-step O-methylation of myricetin to dimethoxy products with high conversion efficiency, and the peak of monomethoxy products can hardly be observed. Possibly due to the chemical equivalence of the 3' and 5' hydroxyl group of myricetin, most plants' 3'-FOMT can simultaneously methylate both 3'-OH and 5'-OH[10,42−45]. However, there are also plant FOMTs that can specifically catalyze the 3 'hydroxyl site of myricetin, such as SlMOMT4 in tomato[46], while Ml08G0855 can catalyze both monomethoxylation and dimethoxylation of myricetin at the same time, which may be related to its specific site selectivity. Ml05G3914 is capable of catalyzing the methylation of luteolin, myricetin, baicalein, quercetin, and caffeic acid, suggesting its significant involvement in the methylation process of flavonoids and lignin in M. lasiocarpa. Due to the absence of reference substances for methylation products, this study was unable to confirm the structure of the product and only verified OMT's function in vitro. Once the structure is identified, further research can be conducted to validate its biological function in vivo and enhance the bioactive components of M. lasiocarpa.
Furthermore, our previous research has demonstrated that Ml01G0494, Ml04G2958, and MI08G0855 are capable of catalyzing the O-methylation of PhPNs, which serve as crucial phytoalexins in bananas and play a pivotal role in defense against pathogenic microorganisms, nematodes, and pests. However, it was found that Ml05G3914 does not possess this capability. Additionally, considering their distinct preferences for flavonoid substrates, this observation prompts further investigation to understand its underlying mechanism. Phylogenetic analysis revealed that Ml01G0494 and Ml04G2958 are closely clustered with high homology, suggesting relatively similar functions - a finding supported by in vitro enzyme activity experiments. The inability of Ml05G3914 to catalyze PhPNs is likely attributed to differences in its substrate binding sites, particularly the substitutions at positions M128Q and V314G. This hypothesis warrants validation through molecular docking and site-directed mutagenesis techniques.
-
In summary, we identified a novel O-methyltransferase, Ml05G3914, belonging to the caffeic acid-O-methyltransferase family. We also characterized the activities of three previously reported OMTs that catalyze the O-methylation of flavonoids: M101G0494, M104G2958, and M108G0855. Specifically, M101G0494, M104G2958, M108G0855, and Ml05G3914 can catalyze the mono-O-methylation of luteolin and caffeic acid, as well as the two-step O-methylation of myricetin. Notably, except for M108G0855, M101G0494, M104G2958, and Ml05G3914 all exhibit the ability to catalyze the mono-O-methylation of baicalein and quercetin. These findings will be crucial for enhancing the nutritional profile of M. lasiocarpa and for advancing enzymatic engineering in the biosynthesis of natural bioactive compounds.
This work was supported by the National Natural Science Foundation of China (Grant Nos 32070360 and 22207047); Natural Science Foundation of Jiangsu Province (BK20220752 and BK20220749), and the Jiangsu Institute of Botany Talent Fund (JIBTF202304).
-
The authors confirm their contribution to the paper as follows: study conception and design: Chen Y, Zhao W, Huang L; data collection: Huang L, Li P, Wu J; analysis and interpretation of results: Wu J, Feng B, Tian M, Xu S, Feng X; draft and revised manuscript preparation: Huang L, Lyu W, Chen Y, Zhao W. All authors reviewed the results and approved the final version of the manuscript.
-
The project number PRJNA1009687 provides access to reference genome data and transcriptome sequence reads in GenBank. The GenBank accession numbers of four MlOMT genes are listed as follows: Ml01G0494 (PQ816233), Ml04G2958 (PQ816234), Ml08G0855 (PQ816235), and Ml05G3914 (PQ816236). The amino acid sequences of four MlOMTs are listed in Supplementary Table S3.
-
The authors declare that they have no conflict of interest.
-
# Authors contributed equally: Long Huang, Pirui Li
- Supplementary Table S1 Primers used in this paper.
- Supplementary Table S2 GenBank accession numbers of OMT proteins from other plants for phylogeny analysis.
- Supplementary Table S3 The amino acid sequences of MlOMTs from M. lasiocarpa.
- Supplementary Fig. S1 Conserved domains of MlOMTs from M. lasiocarpa. (a) Ml01G0494, (b) Ml04G2958, (c) Ml05G3914, (d) Ml08G0855.
- Copyright: © 2025 by the author(s). Published by Maximum Academic Press, Fayetteville, GA. This article is an open access article distributed under Creative Commons Attribution License (CC BY 4.0), visit https://creativecommons.org/licenses/by/4.0/.
-
About this article
Cite this article
Huang L, Li P, Wu J, Feng B, Tian M, et al. 2025. Functional characterization of O-methyltransferases involved in biosynthesis of flavonoids in dwarf banana Musella lasiocarpa. Medicinal Plant Biology 4: e005 doi: 10.48130/mpb-0025-0004
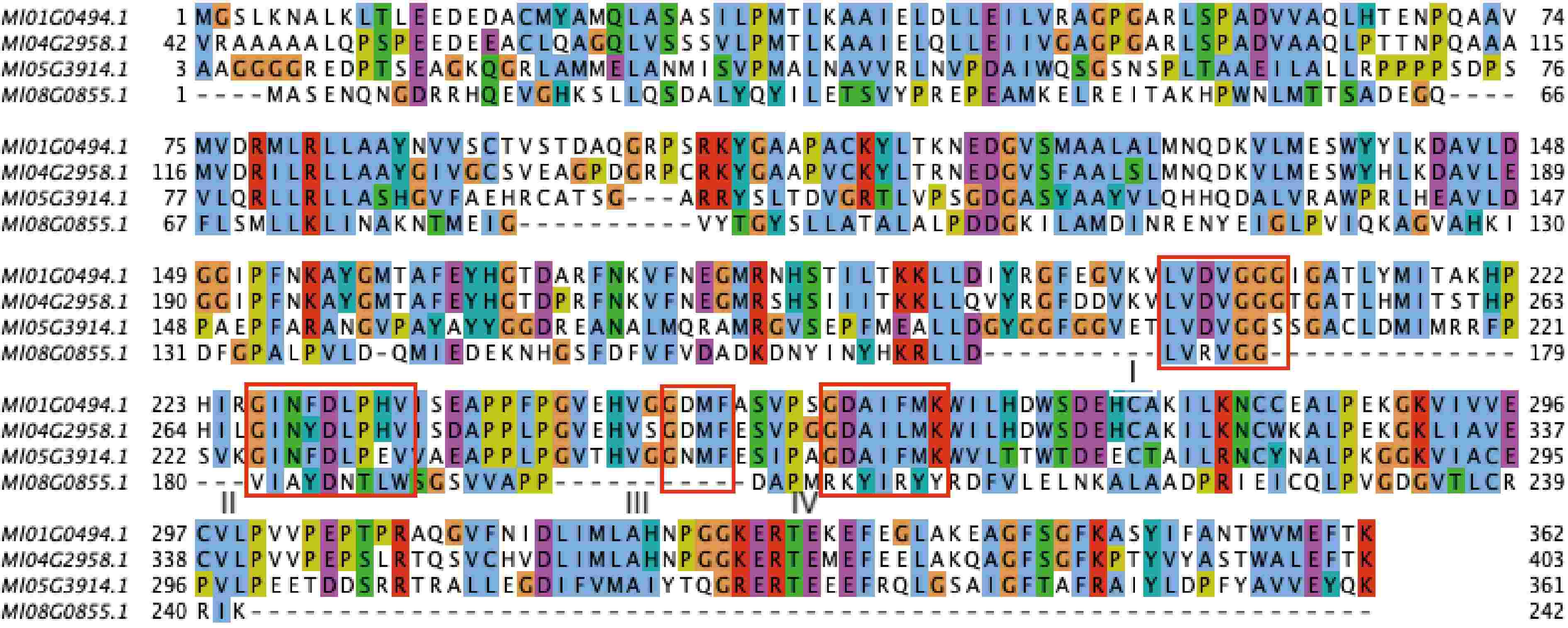