-
Lilium leichtlinii var. Maximowiczii (Regel) Baker, a perennial bulbous herb of the Liliaceae family, is a natural variation of the lemon lily (L. leichtlinii) and is a natural diploid (2n = 2x = 24). L. leichtlinii var. Maximowiczii originated from north-eastern and north-central China, as well as Shaanxi, and it is widely distributed in shrubbery, meadows, and valleys at altitudes of 300−900 m in the Changbai Mountains. This herb has strong cold and shade tolerance and is known as the Changbai Mountain Sweet Lily[1,2]. Owing to its erect stems, pinnate leaves, pendulous orange‒red flowers with dark brown spots on the petals, and curled edges, L. leichtlinii var. Maximowiczii bears a strong resemblance to L. lancifolium lily and can be used as a garden lily or for landscape greening[3,4]. The bulb of L. leichtlinii var. Maximowiczii is globular, with thick, white scales that are more tightly clustered, and it contains a wealth of nutrients; therefore, it has emerged as a new type of edible and medicinal lily in recent years[5]. Promoted and cultivated in Liaoning Province, represented by the Liaoning Academy of Agricultural Sciences in Lingyuan and Fushun, as well as in Dehui, Jilin Province, L. leichtlinii var. Maximowiczii is also used as an edible lily in Japan[6]. Studies have shown that the yield of L. leichtlinii var. Maximowiczii can reach
1,500 kg/mu, which is comparable to that of the traditional edible Lanzhou lily (L. davidii var. unicolor), and its bulbs contain higher levels of protein, starch, vitamin C, total saponins, and total flavonoids than the Lanzhou lily, making L. leichtlinii var. Maximowiczii an excellent raw material for high-nutrition and healthy foods[7]. However, L. leichtlinii var. Maximowiczii has a relatively tall plant height (up to 2–2.5 m), is prone to lodging, and its bulbs spread through roots, necessitating further improvements in its genetic traits to cultivate high-quality, high-yielding new varieties and promote the development and upgrading of the edible lily industry.Colchicine-induced mutagenesis often results in polyploid materials with thickened organs, strong stress resistance, and improved ornamental value, which are highly valuable for the breeding of new varieties of lily. In recent years, polyploid breeding of lilies has become a research focus and has achieved a series of good results[8]. For example, colchicine was used to induce polyploidy in the endemic Tsingtau lily (L. tsingtauense) in China, with an induction rate of 53.3%[9]; colchicine was used to induce chromosome doubling in seeds of the spotted lily (L. concolor var. pulchellum), with the highest induction rate reaching 44.43%[10]; and colchicine was used to induce the lily (L. pumilum) and Lanzhou lily by the soaking method and mixed seedling method, with mutation rates of 48% and 57.14%, respectively[11]. Using colchicine to induce small bulbs of the lemon lily (L. leichtlinii) in vitro resulted in two new tetraploid germplasms with larger flower sizes and earlier flowering[12]. However, to date, research on L. leichtlinii var. Maximowiczii has mainly focused on rapid tissue propagation, karyotype research, field cultivation management, and hybrid selection. Few studies have examined colchicine-induced polyploidy and its application in this species, and no studies have examined the induction of L. leichtlinii var. Maximowiczii by colchicine[2−5,13].
Inter simple sequence repeat (ISSR) is a DNA molecular marker technology based on microsatellites that was developed without relying on genomic sequence information, and it has been successfully applied in the identification and genetic variation analysis of new Lily germplasms[11,14,15]. In previous studies, our group used small bulblets (scales) of L. callosum, L. rosthornii, and Lanzhou lily as materials and successfully cultivated multiple new germplasms via in vitro colchicine induction[16−18]. Considering the significant toxic effects of colchicine on small bulblets during tissue culture, the use of seeds for induction has the advantages of low toxicity, a short period to obtain mutant plants and a high survival rate[19]. In this study, we selected the seeds of L. leichtlinii var. Maximowiczii as materials and used different concentrations of colchicine to induce variations. By combining morphological observations, chromosome ploidy analysis, and ISSR molecular marker identification, we aimed to obtain mutant polyploid materials of L. leichtlinii var. Maximowiczii. These results provide important and valuable germplasms for the development and application of this species.
-
The seeds of L. leichtlinii var. Maximowiczii, a diploid (2n = 2X = 24) plant, were used in the experiment and obtained from the Heilongjiang Forest Botanical Garden.
Seed treatment by colchicine induction
-
Firstly, healthy, pest-free, and normally embryonic L. leichtlinii var. Maximowiczii seeds were selected and soaked in water to promote germination for 2–3 d. Then, colchicine solutions of different concentrations (0.1%, 0.15%, and 0.2%) were prepared with 2% dimethyl sulfoxide (DMSO), and the seeds were soaked in the solutions. The mixture was placed in the dark and shaken for 24, 36, and 48 h respectively. Each treatment group included 60 seeds, and the control group included 60 seeds that were treated with clean water. After colchicine treatment, the seeds were washed with clean water 3–5 times and then directly sown in 9-cm nutrient pots for growth. The photoperiod was set to 16 h/8 h in the illuminated cultivation room, with a temperature of 22–24 °C/day and 16–18 °C/night, and the light intensity was approximately 10,000 lux.
Preliminary screening of mutant seedlings
-
After the treated seeds germinated and the embryonic axes grew, the progress of growth and development was observed every week, and the shapes of the embryonic axes and cotyledons were photographed and recorded at 42 d, which were compared with those of the control group. The seedlings with thickened hypocotyls or malformed or thickened cotyledons were considered mutated seedlings (i.e., seedlings with any morphological changes). The survival rate and morphological mutation rate of all the seedlings were determined.
Observation of morphology in mutant plants
-
According to the growth and development of the mutagenized seedlings, they were transplanted into larger (approximately 18 cm) pots for growth and were subsequently planted in an illuminated cultivation room. Approximately 17 months later, the morphological characteristics of the mutant plants were observed and recorded, and the heights, stem diameters, and leaf sizes of all the mutant plants were measured.
Identification of chromosome ploidy
-
The upper tender leaves of all surviving mutagenized plants were used as materials, and flow cytometry (NovoCyte 2040R, Aisen Biology (Hangzhou) Co., Ltd., Hangzhou, China) was used to analyse chromosome ploidy. Specific operations were performed according to the methods of Chen et al.[20] For chromosome counting, one centimetre of fresh root tips from the mutant plants was used as the material, and the root tip pressing method was used to analyse and identify the number of chromosomes. Specific operations were performed according to the methods of Fu et al.[21].
Comparison of leaf epidermal morphology
-
Fresh leaves from the middle of the No. 2 and No. 3 mutant plants and control plants were removed, and tweezers were used to peel off the leaf epidermis to make temporary slides. The upper and lower epidermal cells and guard cells were observed under an Olympus microscope (BX53, TOKYO 163-0914, Japan) to observe the morphology of the cells. The length and width of more than 60 epidermal cells and guard cells were randomly measured via a microscopic micrometer, and the stomatal density (the number of stomata per square millimetre) was determined.
ISSR marker analysis
-
Using the leaves of the No. 2 mutant plant and the L. leichtlinii var. Maximowiczii control as materials, the genomic DNA was extracted via the CTAB method, 15 ISSR markers were selected for PCR amplification, and agarose gel electrophoresis was used for detection. ISSR marker primers were designed and synthesized according to Sun et al.[11] and ISSR-PCR optimization was performed according to Li et al.[22]. Three independent plants were selected for each ISSR marker primer for analysis. Polymorphic band variation rate (%) = Number of polymorphic bands/Total number of detected bands × 100%.
Statistical analysis
-
Statistical analysis was performed via Excel 2023 and SPSS 27 software. The data are expressed as the mean ± standard error, and significant differences were tested via Duncan's test (p < 0.05).
-
The seeds of L. leichtlinii var. Maximowiczii were treated with different concentrations of colchicine and cultivated in a culture tray. The seedlings emerged at approximately 14 d, whereas those in the control group germinated at approximately 10 d. The uniformity of seedling emergence in the induced group was significantly lower than that in the control group. Forty-two days after sowing, the survival and variation of the seedlings were determined. In total, 236 seedlings survived with a rate of 43.70%. The variant seedlings presented different phenotypes, such as thickened embryo shoots, growth deformities, swollen embryo shoot tips with damaged growing points, shortened and thickened embryo shoots with rough surfaces, increased thickness and deepened colour of the embryo shoots, a droplet shape and slow growth (Fig. 1). The induction effect varied based on concentrations and treatment times. As shown in Table 1, the highest variation rate of the seedlings was 31.67% when the colchicine concentration was 0.15% and the treatment time was 48 h; the second highest variation rate of the seedlings was 30.00% when the concentration was 0.2% and the treatment time was 48 h; and the lowest variation rate of the seedlings was 15% when the concentration was 0.15% and the treatment time was 24 h. When the concentration was 0.10%, the average survival rate of the seedlings was the highest, at 47.22%. With increasing colchicine concentration, the survival rate of the seedlings decreased significantly. Under the same concentration, the increase in the treatment time did not reflect the variation rate of the seedlings among these treatments. While the average rate of 25.56% was achieved in the mutant seedlings (Table 1).
Figure 1.
Colchicine-induced morphological variation in L. leichtlinii var. Maximowiczii seedlings at 42 d. (a) Coarsening and malformed growth of the embryo. (b) Bulging at the tip of the embryo and damage to the growing point. (c) Malformation at the tip of the embryo. (d) Malformation of the lower hypocotyl. (e) Bent and malformed growth of the embryo. (f) Shortening, thickening, and coarsening of the embryo with a rough surface. (g) Imprint. (h) Embryo thickening and darkening in colour. (i) Droplet-shaped. (j) Embryo thickening. (k) Slow growth. (l) Control seedling. Scale bars represent (a)−(k) 8 mm, and (l) 12.8 mm.
Table 1. Effects of colchicine concentration and treatment time combinations on the seeds of L. leichtlinii var. Maximowiczii.
Concentration (%) Treatment time (h) No. of seeds No. of survivors Survival rate (%) Variance Mutation rate (%) 0.10 24 60 31 51.67 17 28.33 36 60 27 45.00 14 23.33 48 60 27 45.00 16 26.67 0.15 24 60 25 41.67 9 15.00 36 60 30 50.00 14 23.33 48 60 26 43.33 19 31.67 0.20 24 60 26 43.33 16 26.67 36 60 21 35.00 15 25.00 48 60 23 38.33 18 30.00 Morphological observation of suspected mutant plants
-
A total of 138 seedlings were obtained from all the treated seeds with colchicine and cultivated under normal conditions in an illuminated cultivation room. Fourty-six water-treated seedlings were used as the controls. The water was changed every week. After 17 months of observation, only 11 of the mutant seedlings survived (numbered No. 1−No. 11), representing a low survival rate of 7.97%. Compared with the control plants, the No. 2 suspected mutant plants presented rapid growth, with the highest plant height at 102.50 cm, and flowering ahead of time (Fig. 2b & Table 2); the No. 3 suspected mutant plants grew slowly, with the shortest plant height of 34.51 cm and much wider leaves of 0.79 cm (Fig. 2c & Table 2). The other plants, which were numbered No. 1 and Nos 4−11, hadheights of 61.32, 48.75, 52.81, 39.74, 40.51, 59.62, 49.51, 39.01, and 55.12 cm, respectively. These heights were lower than those of the control plants (68.62 cm) (Fig. 2a, d−l). Additionally, stem diameter, and perimeter were significantly increased in the No. 2 and No. 8 suspected mutant plants than those in the control plants (Table 2). In addition, Nos 8, 9, and 11 plants presented a branching growth, accompanied by the development of one to two lateral buds (Fig. 2h, i, k). On the basis of these plant phenotypes, it was suggested that the Nos 2, 3, and 8 plants might be real mutant plants.
Figure 2.
The plant morphology in suspected mutant plants after growth for 17 months in the greenhouse. (a)−(k) No. 1−No. 11 mutant plants. (l) L. leichtlinii var. Maximowiczii control. Scale bars represent (a, h, i, k, l) 11.25 cm, (b) 15 cm, (c, d, f, g, i) 7.5 cm, and (e) 10 cm, respectively.
Table 2. Comparison of morphological indicators in L. leichtlinii var. Maximowiczii and suspected mutant plants.
Sample Plant height (cm) Stem diameter (mm) Stem perimeter (mm) Leaf length (cm) Leaf width (cm) Control 68.62 ± 0.00 b 3.09 ± 0.00 b 9.70 ± 0.00 b 14.98 ± 0.73 bc 0.65 ± 0.03 b No. 1 plant 61.32 ± 0.00 c 3.04 ± 0.00 c 9.52 ± 0.01 c 15.37 ± 0.90 b 0.47 ± 0.04 cd No. 2 plant 102.50 ± 0.00 a 3.31 ± 0.00 a 10.41 ± 0.01 a 18.06 ± 0.97 a 0.54 ± 0.03 bc No. 3 plant 34.51 ± 0.00 i 2.56 ± 0.00 d 8.04 ± 0.01 e 10.83 ± 0.86 de 0.79 ± 0.07 a No. 4 plant 48.745 ± 0.01 g 2.19 ± 0.00 e 6.90 ± 0.00 f 12.75 ± 0.84 bcd 0.65 ± 0.08 b No. 5 plant 52.81 ± 0.01 f 3.10 ± 0.00 b 9.75 ± 0.00 b 11.95 ± 0.46 d 0.59 ± 0.05 bc No. 6 plant 39.74 ± 0.01 h 2.60 ± 0.00 d 8.19 ± 0.01 d 10.72 ± 0.96 de 0.41 ± 0.02 d No. 7 plant 40.51 ± 0.01 h 3.05 ± 0.00 c 9.60 ± 0.00 c 12.91 ± 0.43 bcd 0.55 ± 0.03 bc No. 8 plant 59.62 ± 0.00 d 3.31 ± 0.00 a 10.40 ± 0.01 a 13.26 ± 0.81 bcd 0.55 ± 0.04 bc No. 9 plant 49.51 ± 0.01 g 2.09 ± 0.00 f 6.61 ± 0.00 g 8.63 ± 0.68 e 0.63 ± 0.02 b No. 10 plant 39.01 ± 0.01 h 2.20 ± 0.00 e 6.93 ± 0.00 f 12.79 ± 0.98 bcd 0.54 ± 0.08 bc No. 11 plant 55.12 ± 0.01 e 3.11 ± 0.00 b 9.78 ± 0.01 b 12.35 ± 0.76 cd 0.64 ± 0.02 b The data in each column are mean ± standard error, different letters represent significant difference using Duncan's test (p < 0.05). Identification of chromosome ploidy in suspected mutant plants
-
To confirm the chromosome ploidy of the mutant plants, young leaves were selected from the Nos 1−11 plants for flow cytometry analysis, with normally grown diploid leaves used as controls. The ploidy was judged according to the fluorescence intensity X-Mean of the samples, with the diploid peak set at 20, the tetraploid peak at 40, and the aneuploid peak at 20−40. Among them, the No. 3 plant displayed one peak at a relative fluorescence intensity value of about 30, which was speculated to be aneuploid (Fig. 3b), whereas the No. 2 plant with the early-flowering phenotype showed only one peak at a relative fluorescence intensity value of 20, which means that it might be a diploid (Fig. 3c). The remaining plants, No. 1 and Nos 4−11, presented one peak at a relative fluorescence intensity value of 20, were belonged to diploid plants, which was consistent with the results for No. 2 and the control (Fig. 3a).
Figure 3.
Analysis of chromosome ploidy in mutant plants by flow cytometry. (a) Diploid control. (b) An aneuploid polyploid. (c) Diploid plant. The values of the horizontal axis corresponding to the peaks are labeled clearly to indicate their ploidy level.
Analysis of chromosome number in 11 numbered plants was performed via the classic root-tip pressing method. The results revealed that the chromosome number of the control was 24 (Fig. 4a); the chromosome number of the root tip cells in the No. 3 plant was approximately 38, indicating the aneuploid doubling (Fig. 4d); the chromosome number in the No. 2 plant root tip cells was 24 (Fig. 4c); and the remaining plants (i.e., Nos 1, 4−11), all of which were diploid, also had a chromosome number of 24. These results were consistent with those of the flow cytometry analysis.
Figure 4.
Analysis of chromosome numbers of root tip cells in suspected mutant plants. (a) Control. (b) No. 1 mutant plant. (c) No. 2 mutant plant. (d) No. 3 mutant plant. (e)−(l) No. 4−No. 11 mutant plants. Scale bars represent 20 μm.
Characterization of the leaf epidermis of No. 2 and No. 3 mutant plants
-
The leaves from the same stages were used as materials, and the upper and lower epidermal cells were 496.63 and 300.98 μm in size, respectively, in the No. 2 plants, which were significantly greater than those of the control plants (259.49 and 218.98 μm, respectively), the guard cells were 96.66 μm in length, much longer than those in the control (Fig. 5 & Table 3). In addition, in the No. 3 plants, both the epidermal cells and guard cells were significantly greater than those in the control, and the stomatal density was significantly lower, which was in accordance with the leaf characteristics of the polyploid plants. These results further confirmed that the variations in the No. 2 and No. 3 plants were induced by colchicine.
Figure 5.
The observation of leaf epidermal cells and stomatas in No. 2 and No. 3 mutant plants. (a)−(c) The upper epidermal cells in the control of L. leichtlinii var. Maximowiczii, No. 2 and No. 3 mutant plants respectively. (d)−(f) The stomatas of the lower epidermis in the control, No. 2 and No. 3 mutant plants respectively. Scale bars represent 50 μm.
Table 3. Comparison of leaf epidermis, guard cells, and stomata in L. leichtlinii var. Maximowiczii, No. 2 and No. 3 mutant plants.
Sample Adaxial cell
length (μm)Adaxial cell
width (μm)Abaxial cell
length (μm)Abaxial cell
width (μm)Guard cell
length (μm)Guard cell
width (μm)Stomatal frequency
(No./mm2)Control 259.49 ± 4.79 a 51.97 ± 1.25 a 218.98 ± 4.70 a 53.14 ± 0.65 b 78.81 ± 0.53 a 51.01 ± 0.17 b 59.36 ± 0.56 a No. 2 plant 496.63 ± 8.57 b 54.52 ± 1.10 a 300.98 ± 10.68 b 47.36 ± 0.86 a 96.66 ± 0.63 b 46.97 ± 0.16 a 46.19 ± 0.82 b No. 3 plant 472.53 ± 22.41 b 66.90 ± 2.43 b 317.49 ± 11.97 b 55.06 ± 1.32 b 92.16 ± 0.47 b 50.58 ± 0.20 b 35.97 ± 1.21 c The data are mean ± standard error, different letters represent significant difference using Duncan's test (p < 0.05). Identification of the No. 2 mutant plant via the ISSR marker
-
To further identify the early-flowering variation of No. 2 plants, 15 ISSR primers were selected for conventional PCR amplification to observe the polymorphic bands. The results revealed that nine ISSR primers, i.e., 3A26, 3A37, 3A50, 3A59, UBC814, UBC835, UBC840, UBC880, and UBC895, showed no significant differences in the amplified bands between the control and No. 2 mutant plants. However, six ISSR primers, i.e., UBC815, UBC820, UBC825, UBC844, UBC845, and UBC857, showed polymorphic differences in the amplified bands (Fig. 6). On the basis of the ISSR-PCR results, 216 and 207 bands were detected in the control plants and No. 2 plants, respectively, with averages of 4.8 and 4.6 bands amplified by each primer, respectively. No variant bands were observed in three replicate samples each. Compared with those of the control plants, the ISSR primer amplification bands of the No. 2 plants significantly changed. Among the 79 bands detected, 15 polymorphic difference bands were detected, and the ISSR band variation rate was approximately 18.99%. This result suggested that the No. 2 plant was a real mutant line induced by colchicine.
Figure 6.
ISSR detection for No. 2 early flowering plant from L. leichtlinii var. Maximowiczii. (a) Primer UBC815. (b) Primer UBC820. (c) Primer UBC825. (d) Primer UBC844. (e) Primer UBC845. (f) Primer UBC857. 1−3: Three independent samples of No. 2 mutant plant. 4−6: Three independent control samples; M: Marker 5,000 (bp).
-
L. leichtlinii var. Maximowiczii, a new edible lily, is widely grown in north-east China and holds considerable economic value. However, L. leichtlinii var. Maximowiczii plants are usually too tall, and the bulbs undergo stem walking; thus, their quality urgently needs to be improved. Polyploid breeding can be used to cultivate new germplasms with thickened organs and enhanced resistance, but there are no reports on the breeding research of L. leichtlinii var. Maximowiczii induced by colchicine[8]. In this study, we attempted for the first time, to induce variation in L. leichtlinii var. Maximowiczii via in vitro colchicine, to obtain high-quality and high-yielding new lily germplasms to compensate for market deficiencies. In the study of polyploid lily induction, most tissues, such as bulblets (or scales), cluster shoots, and embryogenic calli, have been used; however, few studies have focused on using seeds[8]. Herein, we chose to use L. leichtlinii var. Maximowiczii seeds as the material, and we designed a gradient treatment of three concentrations of colchicine and three different periods. The results revealed that, as the concentration of colchicine increased, the variation rate did not follow a clear pattern. This finding differed from those of previous studies on the induction of Lanzhou lily[18] and L. callosum[23], wherein the former, the variation rate first increased but then decreased, and in the latter, it gradually increased. This may be related to different genotypes or treated materials. Ultimately, treating L. leichtlinii var. Maximowiczii seeds with 0.15% colchicine for 48 h resulted in the best induction effect, with a variation rate of 31.67%. This result was consistent with the mutant effect of colchicine on L. callosum seeds, with a variation rate of 30.00% reported by Yang et al.[19], which was slightly lower than the variation rate of L. concolor var. Pulchellum (44.43%) reported by Zhang et al.[10].
Colchicine is highly toxic and can cause certain toxic effects on embryos during the treatment process. After colchicine treatment, the L. leichtlinii var. Maximowiczii seeds germinated later than those in the control group, and the survival rate of the L. leichtlinii var. Maximowiczii seedlings decreased with increasing colchicine concentration. During the growth and development of mutant seedlings, most of them presented specific characteristics, such as wider leaves, darker colour, and slower growth, which are consistent with suspected mutant lily polyploids[11,24]. However, after 17 months of cultivation, only 11 mutated plants survived, and the survival rate decreased significantly to only 7.97%. Firstly, the reason was possibly related to the environmental factors, such as lack of ventilation in our greenhouse; and secondly, over-watering might lead to the humid soils, that was not suitable for growth of the lily bulblets; in addition, here the death of lily mutant plants might partly be due to the harm of colchicine induction in the seed germination stage. It is known that colchicine could interact with DNA to induce gene mutation by groove binding, and also cause chromosome deletion and translocation, resulting in gene fragment loss and gene variation[25,26]. The cell metabolism during the seed germination stage were more vigorous and prone to mutation by colchicine[10]. Thus, we suspected that some vital genes might be mutation or loss for the growth and development of mutant plants in the later stage.
Morphological observations revealed that induced No. 2 mutant plants presented faster growth, that is, taller plants and earlier flowering, similar to the mutant JD-h-15 line from L. lancifolium, whose plants were also taller and had a 2-week earlier flowering period[21]. Subsequent observations revealed that the control plants started to flower at 23 months, indicating that the No. 2 mutant plant had flowered for about 6 months, preliminarily as an early-flowering variant plant. The induced No. 3 plants presented significant dwarfing, wider leaves, and larger epidermal cells and guard cells with a significant decrease in stomatal density, which was similar to the characteristics of polyploids[11,12]. In addition, the No. 8 plants exhibited dwarfing and increased stem diameter. The other plants presented slow growth or reduced plant height, and these phenotypes might be related to the damages caused by colchicine.
In previous studies, tetraploid, chimeric, and aneuploid plants were usually obtained via the use of colchicine to induce polyploidy in lilies, but there have been few reports of obtaining mutant plants with the same ploidy[9,11,27]. In this study, flow cytometry analysis of 11 suspected mutated plants revealed that only the No. 3 plant was aneuploid, whereas the other induced plants belonged to diploid plants, consistent with that of the control. The squashing of the root tip cells further confirmed the above results: the chromosome number of the No. 3 plant was approximately 38, and the remaining plants all had 24 chromosomes, similar to the control. However, the No. 2 plant showed rapid growth and early flowering, and it was speculated that this variation phenotype was not related to chromosome doubling but may have been due to gene deletion or mutation caused by colchicine. Studies have shown that the molecular structure of colchicine is similar to that of the base molecules of genes, which can cause mispairing of bases to induce gene mutation, or that colchicine can form insertions that bind and grooves that bind with DNA to induce gene mutation[25,28]. In our results, no tetraploid or chimeric plants were detected, possibly because the survival rate of induced plants was too low (7.97%) during long-term culture, and polyploid plants did not survive.
ISSR molecular marker technology is characterized by high polymorphism, genetic stability, and insensitivity to cultivation conditions and environmental changes and has been widely used in the identification of mutant plants[29]. Keykha et al.[14] first used ISSR markers to identify lily mutants and reported that they were efficient at detecting genetic variations, which was beneficial for the early selection of mutants. Guo[30] used ISSR marker technology to identify three salt-tolerant mutants of Lanzhou lily. Cai et al.[31] used ISSR markers to identify early-flowering mutants of the cut flower chrysanthemum variety 'Shenma'. In this study, an ISSR molecular marker was used to analyse and identify No. 2 mutant plants, and the results revealed that, compared with those of the control, the PCR products of the six ISSR primers were polymorphic, with varying sizes of different sites, and the total genetic variation rate was 18.99%. Therefore, it was speculated that the early flowering of the No. 2 mutant plant was not due to changes caused by the environment but rather was due to gene mutation. In summary, two valuable mutant plants of L. leichtlinii var. Maximowiczii, namely, No. 2, the early flowering mutant plant, and No. 3, the aneuploid plant, were ultimately obtained via colchicine induction in this study. This work provides a basis not only for the cultivation of high-yielding and superior-quality varieties of L. leichtlinii var. Maximowiczii but also for screening mutant genes and further explaining the mechanism of the early-flowering phenotype in lily.
-
Treatment of the seeds of L. leichtlinii var. Maximowiczii with colchicine for 48 h resulted in the most effective induction, with a morphological variation rate of 31.67% in the seedlings. After being cultured for 17 months, 11 suspected mutant plants were obtained, and compared with the control plants, the No. 2 plants grew rapidly and flowered earlier, and the No. 3 plants were significantly dwarfed and exhibited wider leaves. Chromosome ploidy analysis revealed that the No. 2 plant was diploid, and the No. 3 plant was aneuploid, with 24 and 38 chromosomes in the root tip cells, respectively. Microscopic observations revealed that the leaf epidermal cells and guard cells were enlarged in the No. 3 plant and that the stomatal density was significantly reduced, which is consistent with the characteristics of polyploid plants. Compared with the control, the ISSR markers revealed genetic variation in No. 2 plants at the molecular level, which was a new mutant with an early-flowering phenotype. This study revealed two important mutant materials, providing the basis for further research on the mechanism of early flower formation and the cultivation of superior varieties of lily.
This work was supported by the Technology Innovation and Application Development Program of Fuling Science and Technology Bureau (FLKJ,2023AAN1001), Natural Science Research Program of Chongqing Science and Technology Bureau (cstc2019jcyj-msxmX0014) and the Research Funding Program of Yangtze Normal University (2017KYQD63). The authors thank Doctor Sijia Jiang in Heilongjiang Forest Botanical Garden for supplying the seeds of Lilium leichtlinii var. Maximowiczii.
-
The authors confirm contribution to the paper as follows: study conception: Fu Y; data curation: Fu Y, Xu W; investigation: Fu Y, Ren J, Guo R, Lu X, Lei Y; methodology: Fu Y, Xu W; supervision: Fu Y; writing – original draft: Fu Y, Ren J; writing – review & editing: Fu Y. All authors reviewed the results and approved the final version of the manuscript.
-
The datasets generated during and/or analyzed during the current study are available from the corresponding author on reasonable request.
-
The authors declare that they have no conflict of interest.
-
# Authors contributed equally: Yongyao Fu, Jiajia Ren
- Copyright: © 2025 by the author(s). Published by Maximum Academic Press, Fayetteville, GA. This article is an open access article distributed under Creative Commons Attribution License (CC BY 4.0), visit https://creativecommons.org/licenses/by/4.0/.
-
About this article
Cite this article
Fu Y, Ren J, Guo R, Lu X, Lei Y, et al. 2025. In vitro induction and molecular and cellular identification of mutant plants of Lilium leichtlinii var. Maximowiczii (Regel) Baker by colchicine. Ornamental Plant Research 5: e014 doi: 10.48130/opr-0025-0012
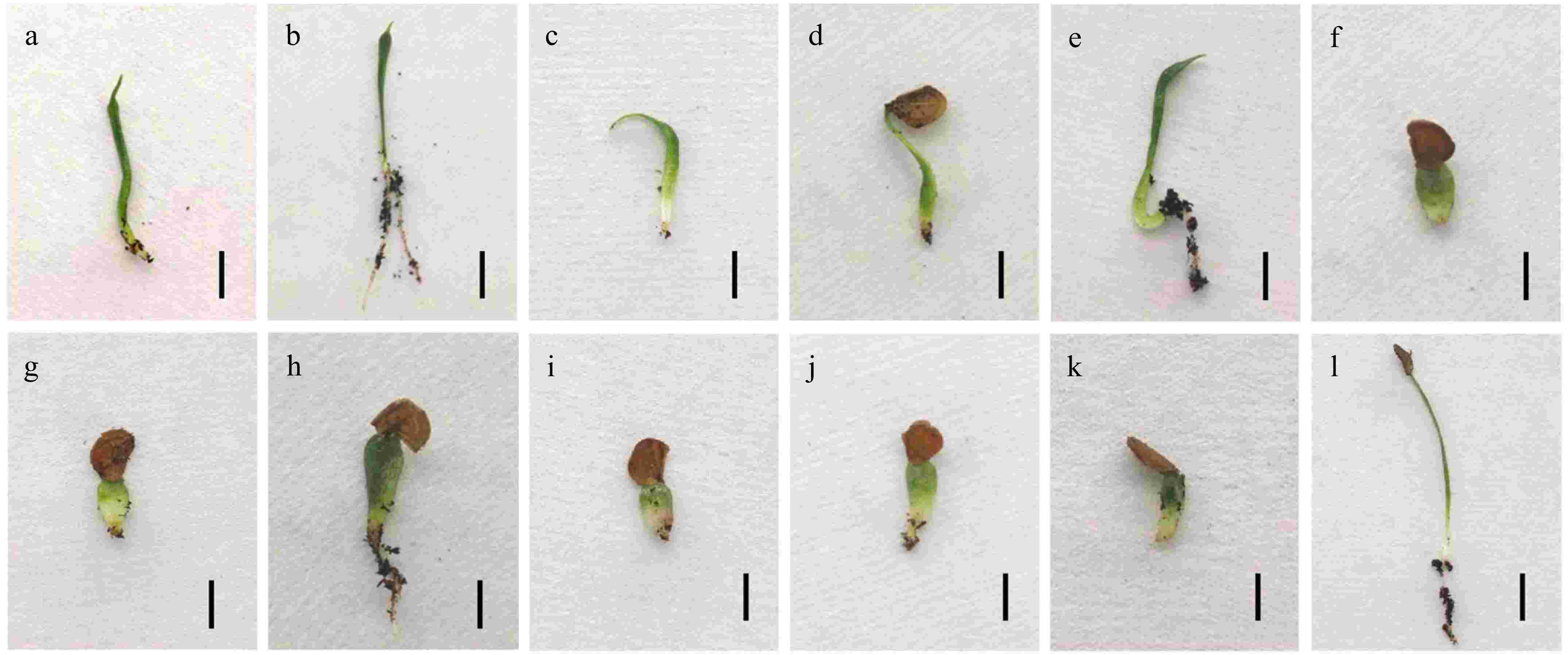