-
Wheatgrass, the young grass of the common wheat plant, contains chlorophyll, flavonoids, and vitamins C and E have a wide range of health benefits[1]. When given as a supplement to standard care, daily wheatgrass juice (WGJ) intake boosted anti-inflammatory cytokine IL-10 concentrations and lowered white blood cell (WBC) counts in colorectal cancer (CC) patients[2]. Five and 7-d wheatgrass extracts significantly inhibit lipopolysaccharide (LPS)-stimulated production of nitric oxide and downregulate LPS-stimulated various pro-inflammatory markers, tumor necrosis factor-α, interleukin-6, interleukin-1β, AP-1, iNOS-2, and COX-2[3]. Wheatgrass significantly upregulated neuroprotective markers such as BDNF and CREB but downregulated the expression of inflammatory markers such as TNF-α and tau genes, hence improving the behavior and memory of amnesic mice[4]. Wheatgrass, or a combination with fluoxetine, alleviates toxic insults such as accumulation of tau protein and Aβ, the elevation of ACHE and GSK-3β, down-regulation of BDNF, and β-catenin expression in Alzheimer's disease (AD) brain induced by AlCl3[5].
Different approaches contribute to enhancing the health-promoting effects of wheatgrass and related products. The wheatgrass extracts showed 1.38−1.67 times higher phenolics, flavonoids, and vitamin C contents than corresponding barley grass extracts and water deficit further improves the antioxidant potential[6]. Lyophilized wheatgrass juice powder (WJLP) from colored wheat had 564.2% higher anthocyanins, 30.5% higher chlorophyll, 87.5% higher soluble phenolic content, 10.2% higher proteins, 31.5% higher EAAI, and 112.5% higher antioxidant activity compared to white WJLP[7]. Wheatgrass germinated under microgravity contained a unique set of bioactive compounds (e.g., pyridoxine, apigenin, and tocopherol, among others) and displayed enhanced cytotoxic effects against normal skin fibroblasts, normal MCF-10, MCF-7, and breast cancer MDA-231 cell lines[8]. Elicitation of wheat grass with arachidonic and jasmonic acid (JA) increased the antioxidant activity but did not qualitatively change the examined phenolic compounds[9].
This work revealed that wheatgrass flavonoids were higher than wheat grain by order of magnitude. The metabolite analyses of 228 model Chinese wheat cultivars identified the major flavonoids as apigenin, luteolin, and chrysoeriol derivates. Supplied at one mM in foods, the flavonoid extract of wheat (FEW) elongated the lifespan of male fruit flies. There were strong positive correlations between the antioxidant potential and contents of luteolin and chrysoeriol derivates. Based on the flavonoid composition, we selected the cultivars with higher flavonoids and confirmed their advantages in terms of antioxidant potential. To further increase flavonoids, we treated the wheat seedlings and detected significant increases in the flavonoid contents and antioxidant potentials. This work expanded our knowledge of the antioxidant potential in wheatgrass by screening high-flavonoid wheat cultivars and JA elicitation, which could enhance the economic value of wheatgrass and benefit consumers' health.
-
Dried seeds were soaked in tap water for 16 h and then placed on wet filter paper a day later, at room temperature, for 1−2 d. The germinating seeds were then transferred to a 4 °C refrigerator upon the appearance of white embryo roots to store them for a week before moving them to the sterilized culture soil. The seedlings were watered regularly during their growth in a 22 °C chamber with a 16-h light/8-h dark cycle. The aerial parts were collected from 1-week seedlings, briefly washed with tap water, and used to extract flavonoids or subjected to JA treatments and RNA extraction.
Extraction of flavonoids
-
All the leaf tissue of the young leaves were cut and put into labeled EP tube for quick freezing with liquid nitrogen. The samples were then homogenized in liquid nitrogen in a tissue grinder (55 Hz, 30 s, twice). One hundred mg of the fine powder was placed into a clean centrifugal tube. 80% methanol at 1 mg was added: 10 μL, the samples were then mixed thoroughly in the tissue grinder (60 Hz, 1 min, twice), and then sonicated for 30 min to extract flavonoids in the leaves. The ultrasonic extract was then centrifuged at 12,000 rpm for 15 min, then the supernatant was carefully transferred to a new centrifuge tube and stored at 4 °C overnight. The liquid was then centrifuged at 12,000 rpm for 15 min, and the supernatant transferred to a new 1.5 mL centrifuge tube with a pipette. The solution was then directly tested or stored in a low-temperature refrigerator at −80 °C for a short storage period of no longer than one month.
Purification of flavonoids
-
YKDH-5 resin was soaked in anhydrous ethanol for at least 24 h to make it fully swell. The soaked resin was then drained into ethanol and immediately rinsed with deionized water until there was no noticeable alcohol smell. The resin was then soaked in 4% sodium hydroxide solution for 3 to 6 h, immediately rinsed with deionized water until neutral. Then the resin was soaked with 4% hydrochloric acid solution for 3 to 6 h, and immediately rinsed with deionized water to neutralize. The pretreated macroporous adsorption resin 1.0, 2.0, and 4.0 g was accurately weighed and placed in 50 mL conical bottles, marked, and mixed with 20 mL wheatgrass extract. Each group had three replicates. The conical bottle was placed in a constant temperature environment at 28 °C and thoroughly shaken at 150 rpm for 12 h for adsorption. After vacuum filtration, the macroporous resin was thoroughly washed with 100 mL of deionized water. After vacuum filtration, 20 mL 50% ethanol was added into the conical bottle and placed on a constant temperature shaking table at 28 °C at 120 rpm for 2 h to elute. The crude extract of flavonoids from young wheat leaves was obtained with 70% ethanol as eluent at 3 BV·h−1 for 1 h. The samples were dried in a rotary dehumidifier at 200 rpm at 40 °C. The cooling was carried out with a −10 °C cryogenic coolant circulation pump. The crude extract of wheat flavonoids was speed-dried and stored at low temperature.
Characterization of flavonoids
-
The samples were injected into a column at 30 °C, with the flow rate at 0.8 mL·min−1 and the detection wavelength at 340 nm. The UHPLC-MS system included an Agilent 1290 ultrahigh-performance liquid chromatography system (UHPLC) coupled with an Agilent 6530B Accurate-Mass q-TOF (UHPLC-qTOF-MS) (Agilent Technologies, Inc., USA). Mass analysis data was conducted in the positive ion mode (ESI+) and acquired and MS spectra with a spectral mass-to-charge ratio range (m/z) of 110−950. All the MS spectra were processed using the software package Qualitative Analysis and Profinder (Agilent Technologies, Inc., USA). Mass spectrometry was performed in positive ion mode using the parameters: capillary voltage: 4,000 V; breakage voltage: 175 V; nozzle voltage: 500 V; cone hole body voltage: 65 V; Rf voltage: 750 V; atomizer pressure: 50 psi; dry gas temperature: 350 °C; air curtain temperature: 350 °C. Nitrogen worked as a curtain gas and dry gas flow rates were set at 12.0 L·min−1 and 10.0 L·min−1 respectively. The data was collected in centroid mode, and the impact energy was set to 0, 10, 20, and 40 V using the extended dynamic range. Using MassHunter software, flavonoids in the sample solution were analyzed according to retention time, UV absorption spectrum, and mass spectrometry[10]. Quantification of the compounds were conducted in Thermo Scientific UltiMate 3000 HPLC system (Fisher Scientific Inc. Germany).
Antioxidant activity analysis
-
To prepare a stock solution, 1,1-diphenyl-2-picrylhydrazyl radical (DPPH) was first dissolved in 80% methanol at 10 mM and then the stock solution diluted with 80% methanol until the OD517 equals 0.5 to prepare the DPPH working solution. Ten microliters of samples (standard, soluble, and wall-bound phenolic) were mixed with 190 μL of DPPH working solution in the dark. The samples were mixed for 10 s every 5 min at RT for 30 min. The absorbances at 517 nm of the samples were recorded to calculate corresponding DPPH radical scavenging activity according to the standard curve ranging from 50 to 600 μM with 80% methanol as the negative control[11].
A colorimetric method was applied to quantify the 2,2'-azino-bis (3-ethylbenzothiazoline-6-sulfonic acid, ABTS)-removing activity. The ABTS reagent (7 mM ABTS and 2.45 mM K2S2O8, both in 5 mM PBS, pH 7.4) was prepared in the dark overnight and diluted with 5 mM phosphate buffer (PBS) to OD734 equals 0.7 ± 0.02[11]. A volume of 195 μL ABTS reagent was mixed with 5 μL of crude extracts in a transparent 96-well plate and the plate kept at 37 °C for 5 min. The absorbance at 734 nm was quantified using 80% methanol as the blank and PBS as the negative control. Authentic luteolin and ferulic acid ranging from 50 to 600 μM worked as the standard to build the standard curve[12].
The Ferric ion-reducing antioxidant power (FRAP) working solution contained 300 mM pH 3.6 acetate buffer, 10 mM TPTZ, and 20 mM FeCl3 at a ratio of 10:1:1 (v/v)[13]. The FRAP solution (190 μL) was mixed with 10 μL of the samples (standard, soluble, and wall-bound phenolic) in a transparent 96-well plate and the plate kept at 37 °C in the dark for 30 min with a 10-s shake every 5 min. The absorbance at 593 nm was measured to calculate the FRAP radical scavenging activity according to the standard curve ranging from 50 to 600 μM with 80% methanol as the negative control.
Gene expression analysis by quantitative reverse transcript polymerase chain reaction (qRT-PCR)
-
Total RNA was extracted in developing grains at the milky stage collected from the field using TRIzol (RNAiso Plus, TAKARA BIO INC, Japan). Reverse transcription was performed with 3 μg of total RNA using a Hifair® ІІ 1st Strand cDNA Synthesis Kit (Yeasen, Shanghai, China) according to the user manual. RT-PCR was applied using 2xHieff® Robust PCR Master Mix (Yeasen) with wheat Actin gene as the endogenous control to analyze the gene expression level. Gene-specific primers (Supplemental Table S1) were used to investigate the differential expressed genes. Gene expression was quantified using a comparative threshold of 2−ΔΔCᴛ method. At least three biological replicates were analyzed for each sample.
Fruit fly culture
-
The fruit fly culture medium components were weighed and the mixture stirred on a magnetic stirrer until the brown sugar dissolved. The crud food was heated on an electric stove, and stirred with the medicine spoon to prevent the bottom from burning. After heating to boiling, it was kept on the boil for 1 min and then the heating was stopped. The food was stirred with a medicine spoon until it cooled to 50 °C; 2,450 mg of dry yeast powder was added and stirred until it had completely dissolved. Flavonoids or vitamins were added to the food when it cooled to 40 °C and it was then divided it into culture bottles with syringes, 3 mL/tube, number, and date. A layer using sterile gauze was laid on the tube mouth, and after standing for 12 h, the tube mouth was sealed with an ethanol-sterilized sponge and stored in the refrigerator at 4 °C. The shelf life of the food was one week.
Twenty female or male fruit flies (Drosophila melanogaster, W118) were placed in each culture tube. A total of 10 tubes were collected, numbered, marked with the date, and placed in the incubator. The culture was cultured at room temperature (25 °C). The photoperiod was set to 12 h light and 12 h dark. The incubator was wiped with 75% ethanol every 3 d for disinfection. The media was changed every 2−3 d, and the number of dead flies counted.
-
To expand the supply of antioxidants for human beings, the antioxidant potentials of wheat flavonoids were compared with vitamin C (Vc), one of the most common antioxidant supplements. All four flavonoids showed higher antioxidant potential than Vc in the 2,2'-azino-bis (3-ethylbenzothiazoline-6-sulfonic acid) (ABTS) assay (Fig. 1a). However, in the 1,1-diphenyl-2-picrylhydrazyl radical (DPPH) assay, the DPPH clearance rates of isoorientin and luteolin were around two folds of Vc, but those of isovitexin and apigenin were negligible (Supplemental Fig. S1). The ferric-reducing antioxidant power (FRAP) method showed a similar trend to DPPH (Supplemental Fig. S1).
Figure 1.
Analysis and products of flavonoids in wheat accessions. (a) Comparison of the antioxidant activity of wheat flavonoids with vitamin C using the DPPH method. (b) The contents of flavonoids in grains and wheatgrass on the accessions. Data represent the mean ± standard error. The student’s t-test determined the p-value (hereafter). (c) The phylogenetic relationships of bred history. Ovals represent breeding parents, rectangles represent bred cultivars, and related cultivars are linked by black lines. Ellipse color and variety size deepen and increase with breeding usage. (d) The distribution of the wheat accessions. The size of the circle indicates the number of cultivars. (e) The ancestral information of accessions. The yellow bar chart on the left indicates the number of genes associated with each trait. The black circle and line indicate the overlap groups and the corresponding bar chart shows intersecting gene numbers.
The average flavonoid contents in 243 modern Chinese wheat accessions were only 34.89 ng·g−1 DW in grains (Fig. 1b), significantly lower than wall-bound phenolics[10]. Nevertheless, the flavonoid contents in wheatgrass were around 675 ng·g−1 FW, almost 20 folds in grains (Fig. 1b, Supplemental Table S2). Therefore, wheatgrass has the priority of serving more flavonoids for consumers.
The number of cultivars authorized in the past decade accounted for 82.46%. In terms of parental selection, 18 cultivars appeared over five times, the top four were Zhoumai16 (ZM16, 43 times), Bonong AK58 (AK58, 33 times), Zhoumai22 (ZM22, 19 times), Zhoumai18 (ZM18, 15 times), and the other 14 cultivars 5~8 times (Fig. 1c). The above 228 modern Chinese wheat accessions were bred in the Yellow and Huai River Valleys Facultative Wheat Zone in China (Fig. 1d).
Based on the breeding procedure, 122 wheat cultivars contain Yanshi4Hao (YS) background, and 119 wheat cultivars contain Yumai2Hao (YM3) background. One hundred and nine wheat cultivars have a Yumai21 (YM21) genetic background. There were 109 wheat cultivars containing Zhou 8425B background, of which about 90.83% (99 cultivars) were cultivated by the progeny of Zhenmai16 (ZM16), AK58 (cultivated by Zhoumai11) and ZM22 (Fig. 1c). In addition, 84.40% (92) of the wheat cultivars grown with Zhou8425B contain a YM21 background (Fig. 1e).
The effect of wheatgrass on the lifespan of fruit flies
-
YKDH-5 macroporous adsorption resin has a good adsorption effect on flavonoids in young wheat leaves. The 1, 2, and 4 g of pretreated macroporous resin showed adsorption rates above 99.5% after 12 h of adsorption of 20 mL wheatgrass juice (Fig. 2a). Ethanol at 70% and 50% could completely elute the adsorbed flavonoids, while 30% ethanol only had a 77.0% elution efficiency (Fig. 2b). In terms of the types of glycosylation modification, the adsorption capacity of the macroporous adsorbent resin was hexasaccharide modification > hexasaccharide - deoxyhexose modification > hexose glycosylation modification.
Figure 2.
Purification and animal effects of flavonoids from wheatgrass. (a) Effect of solid-liquid ratio and (b) elution ethanol concentration on static purification of flavonoids by macroporous adsorption resin. (c) The lifespan of male fruit flies fed with foods containing 1 mM of FEW or without (control). (d) The Gene Ontology (GO) analysis of enrichments of differentially expressed genes (DEGs) in male fruit flies fed with FEW or control foods.
To evaluate the health-benefiting effect of FEW, the crude extracts were added into the food and fed to fruit flies. FEW had no apparent impact on the lifespan of female flies (Supplemental Fig. S2). Nevertheless, FEW displayed dose-dependent and gender-specific benefits on the lifespan of male fruit flies, peaking at 1 mM (Fig. 2c, Supplemental Fig. S2). The supplication of FEW did alter some male-specific biological processes, e.g., sperm competition (Fig. 2d). Among the molecular functions, the FEW in foods affected several malate metabolism pathways and thiamine transportation pathways (Fig. 2d). Therefore, energy metabolism and vitamin absorption could be associated with lifespan elongation in male fruit flies.
Identification of the major flavonoids in wheatgrass
-
The flavonoids from wheat seedlings were isolated and identified by UPLC-DAD-MS/MS. Ten major flavonoid glycosides were determined based on the mass spectrum and comparisons with reported flavonoids (Fig. 3a−j). According to aglycones, Peak1, Peak3, and Peak4 were luteolin glycosides. Peak2, Peak5, Peak7 and Peak9 were apigenin glycosides. Peak6, Peak8, and Peak10 are chrysoeriol flavonoids.
Figure 3.
Chemical structures and cleavage modes of major flavonoids in wheatgrass. (a)−(j). The chemical structure and cracking patterns of 10 flavonoids. Top, the MS spectrum of the compound. Bottom, the MS/MS spectrum. (k) Chromatogram of flavonoids in wheatgrass. The black line shows flavonoids in cultivar Z208. The red and blue lines showed authentic isoorientin and isovitexin standards, respectively.
There were apparent rules among the 10 compounds considering their glycosylation modifications. The simplest compounds had mono-saccharylation modifications, such as Peak4, Peak9, and Peak10. They had a 6-glucoside modification and were identified as isoorientin, isovitexin, and isoplantagin, respectively (Fig. 3k). Peak9 and Peak10 had second glycosylation to form Peak5 and Peak6, respectively. Peak10 had another rhamnose-glycosylated modification to form Peak8. Among the compounds, the 6th and 8th positions of the former aglycone could be modified with arabinose at the 6th position or glucose at the 8th position.
Association of the flavonoids with the antioxidant potentials
-
Nineteen leaf increased flavonoid (lif) and 21 leaf reduced flavonoid (lrf) cultivars were selected from the 228 accessions for the second round of testing. In addition, another 22 cultivars worked as controls, whose flavonoids were between 0.8 and 1.3 times the average value (Fig. 4a). Overall, there was a strong correlation between total flavonoid content and the scavenging ability of three free radicals and eight kinds of flavonoid glycosides (Fig. 4a). However, there was no correlation between total flavonoid content and api-hex-pen and api-dehex-hex, nor between api-hex-pen and other compounds or antioxidant capacity (Fig. 4a). The correlation between the various flavonoids in this population was more likely dependent on the type of aglycone. In contrast, the correlation between C-hex and C-hex-hex was more robust within the same aglycone (Fig. 4a).
Figure 4.
Detection and analysis of flavonoids in wheatgrass of modern Chinese wheat accessions. (a) The correlation analysis among various traits in the wheat accessions. The red color indicated a positive correlation between the two traits, while the blue indicated a negative correlation between the two traits. The black number presented R value. * represents the confidence test of correlation analysis. (b) The distribution of cultivars in the principal component analysis. lif, leaf increased flavonoids; lrf, leaf reduced flavonoids. (c) Heat map analysis of flavonoid contents in selected cultivars. (d)−(g) Determination of total flavonoid contents and antioxidant capacities of selected cultivars.
Next, the samples were checked using a principal component analysis. The first principal component of the composite component analysis could explain nearly half of the variance, and the second principal component could explain 20.4% of the variance (Fig. 4b). All the low-flavonoid populations were in the negative region, while all the high-flavonoid populations were in the positive region. In the second principal component, both the low flavonoid group and the high flavonoid group showed some differences, but the difference of the low flavonoid group was more prominent. The results indicated significant differences in the composition of flavonoids in young wheat leaves between the lif and lrf groups, and there were also some differences within each group.
A clustering of the above data divided the 11 characters into two categories, in which the total flavonoid content, four apigenin flavonoids, and chr-dehex-hex fell into one category, and the other two chrysoeriol flavonoid glycosides and three luteolin flavonoid glycosides formed another category (Fig. 4c). A clustering analysis divided the 42 cultivars into six categories. The 16 lrf cultivars formed two categories: seven cultivars lost most of the flavonoid glycosides, except api-hex-pen; while the remaining nine lrf cultivars lost more api-hex-pen (~50%) than others (by about 30%). The lif cultivars usually contained more luteolin and chrysoeriol flavonoids (Fig. 4c).
Based on the principal component analysis and heat map analysis of flavonoids, we selected some representative cultivars for subsequent experiments. Z208 (Xiaoyan 269, XY269), Z182 (Suixuan 101, SX101), and Z217 (Zhoumai 30, ZM30) stood for the high flavonoid wheat population (Fig. 4d). The total flavonoid contents of these three cultivars were higher, and the antioxidant potentials were also significantly increased compared to JM22 (Fig. 4e,f). These three cultivars had high total flavonoid contents and similar compound compositions. Therefore, they possessed the potential as breeding parents to increase the total flavonoid content of wheat leaves and could increase the contents of luteolin and chrysoeriol flavonoid glycosides in offspring (Supplemental Fig. S3) while not reducing the content of apigenin flavonoid glycosides.
Flavonoid metabolism of wheatgrass elucidated with JA
-
Studies have reported that JA can affect the accumulation of flavonoids by activating the phenylpropanoid metabolic pathway[14]. The transcriptomes of JA-treated wheat seedlings were analyzed and the total counts on genes annotated in the flavonoid biosynthesis pathway were calculated. The total expression levels of Phenylalanine lyase (PAL), 4-coumaroyl CoA hydroxylase (C4H), 4-coumaroyl CoA ligase (4-CL), and Chalcone synthetase (CHS) were significantly upregulated by JA (Fig. 5a). The up-regulation of key DEGs were further validated by qRT-PCR, confirming the activation of the phenylpropanoid metabolic flow (PAL, 4-CL, and C4H, Fig. 5b−d) and the branch towards flavonoids (CHS, Fig. 5e).
Figure 5.
Boosting flavonoid biosynthesis by JA treatment. (a) The effect of JA treatment on the wheat flavonoid synthesis pathway. Data were from the NCBI bio project database (PRJNA831434). The red block indicated upregulated in a sample at the whole gene family level. Quantitative RT-PCR analyses of (b) Phenylalanine lyase (PAL), (c) 4-coumaroyl CoA ligase (4-CL), (d) 4-coumaroyl CoA hydroxylase (C4H), and (e) Chalcone synthetase (CHS) genes by JA treatment. (f-i) The changes of (f) total flavonoid contents and (g-i) antioxidant capacities of selected cultivars. n = 4.
To examine the effect of JA, the samples were extracted and quantified the total flavonoids. The JA treatment increased the total flavonoid contents by around 30%, representing a significant increase (Fig. 5f). The JA treatment promoted the antioxidant potential of FEW as measured by FRAP, ABTS, and DPPH methods (Fig. 5g−i). The above data provided a strategy to further enhance flavonoids and the corresponding antioxidant potentials in wheatgrass by exogenous application of JA.
-
Wheatgrass is becoming increasingly popular for its health-promoting effects, including chlorophyll, flavonoids, and vitamins C and E[1]. However, information on the nutrients among different cultivars was limited before this study. This work revealed the composition and contents of flavonoids in a collection of wheat accessions and provided a strategy to increase flavonoids by JA treatment.
Wheatgrass powder (WGP; 3%−15%) significantly enhanced antioxidant activities in terms of DPPH, FRAP, and ABTS, the protein and fiber contents, but 9% WGP-incorporated pasta was optimum based on the sensory results[15]. Using different methods, an exciting and informative finding was gained that luteolin-6-C-glucoside (isoorientin, peak4 in Fig. 3d, k) in wheatgrass had antioxidant activities even higher than vitamin C, which is a widely used antioxidant supplement. Isoorientin can reverse lung cancer drug resistance by promoting ferroptosis via the SIRT6/Nrf2/GPX4 signaling pathway[16]. Therefore, the intense antioxidant activity could be the basis for the benefits of wheatgrass for human health. Moreover, the chemicals with higher antioxidant potential should be the target of some future breeding programs. In the following animal test, a complex effect of flavonoid extract of wheatgrass (FEW) was observed, which prolonged the lifespan of male fruit flies at the 1 mM level in the food (Fig. 2c). The higher concentration, e.g. 1.5 mM, negatively affects the lifespan of male fruit flies (Supplemental Fig. S1). Moreover, FEW had no significant effect on female fruit flies.
Supplemented in rat food, concomitant administration of a 9th-d wheatgrass diet (200 and 400 mg·kg−1) for 60 d significantly improved biochemical indices, including hyperglycemia, body weight, and lipid profile, suggesting wheatgrass as a functional food to control diabetes[17]. Despite the importance of FEW's antioxidant potentials, protein, fibers, and minerals were also reported as health-promoting compositions. The effects of FEW on the lifespans of male or female fruit flies raised the need for appropriate addition concentration ranges of wheatgrass products.
Wheatgrass juice is a natural dietary supplement among many fresh fruit and vegetable juices due to its nutritional value. Compared to other juices, wheatgrass juice had the highest total and in vitro accessible concentrations of Ca, Mg, Mn, Fe, and Zn, and the highest total phenolic and flavonoid content[15]. Black wheat had 87.5% higher soluble phenolic content than white wheat seedlings and 112.5% higher antioxidant activity[7]. Interestingly, except that the total flavonoid content of Yusheng Black 1 was similar to the average content, the total flavonoid content of the young leaves of the other three kinds of color wheat was very low (the last 2%), even though they contained more anthocyanins (Supplemental Table S2). Among the tested modern Chinese wheat cultivars, the contents of flavonoids ranged from 200 to 1,200 mg·g−1 FW (Fig. 1b). Furthermore, the intercrossing between high-flavanoid cultivars created offspring with flavonoid flux towards apigenin or luteolin (Supplemental Fig. S3). The above genetic resources and their future derivated offspring could be promising to dissect the function of different classes of flavonoids and meet the demands of various consumers. Therefore, a proper cultivar selection with wide FEW ranges represents a viable strategy to improve the nutritional value of wheatgrass products.
Sixty two extreme cultivars were then screened from the above accessions and their flavonoid profiling analyzed. Twenty five high-flavonoid cultivars and 16 low-flavonoid cultivars were identified using JM22 as the control (Fig. 4c). Surprisingly, we found that the total flavonoid content of AK58 and its descendent cultivars contained 16% to 33% higher flavonoids than JM 22, suggesting that AK58, one of the top cultivars planted in China, is an excellent high-flavonoid resource. The associations between flavonoids and agronomic traits, such as plant height, breaking force, grain size, and grain weight, were also calculated but failed to produce any clear correlation (data not shown). Hence, genetic engineering FEW in future breeding programs could improve health-promoting functions safely without any adverse effects on yield-related agronomic traits.
-
This work demonstrated that certain flavonoids from wheatgrass had ROS-detoxifying activity comparable to vitamin C. Wheat accessions of 228 modern Chinese contained wide variations of flavonoid compositions and were excellent genetic resources to study the genetic basis and breed new cultivars with higher or specialized flavonoids. The FEW of a high-Flav cultivar successfully elongated the lifespan of male fruit flies at the correct dose, but further investigations are needed to screen future flavonoid-specialized germplasms to identify the combination/concentration benefiting female fruit flies.
We thank Dr. Yanpeng An at Fudan University for his valuable help with the compound analyses. This study was supported by the National Natural Science Foundation of China (32372557), the Development of Mongolia through Science and Technology (NMKJXM202201), and Pinduoduo - China Agricultural University Research Fund (PC2023B02013).
-
The authors confirm contribution to the paper as follows: study conception and design: Guo JY; experiments: Wang CY; gene expression patterns confirmation: Wang ML, Li QQ; draft manuscript preparation: Yan Y, Hao S, Guo JY. All authors read and approved the final manuscript.
-
The data supporting this study's findings are available in the NCBI bio project database (PRJNA831434). These data were derived from the following resources available in the public domain: https://ncbi.nlm.nih.gov/bioproject/831434.
-
The authors declare that they have no conflict of interest.
- Supplemental Table S1 The sequences of primers used in the qRT-PCR analysis.
- Supplemental Table S2 The total flavonoids in grains and seedlings of cultivars used in this study.
- Supplemental Fig. S1 Comparison of the antioxidant activity of wheat flavonoids with vitamin C and rutin using the ABTS and FRAP methods.
- Supplemental Fig. S2 The survival curve of male and female fruit files on foods with different concentrations of FEW.
- Supplemental Fig. S3 The offspring of two high flavonoid cultivars with different compositions of flavonoids.
- Copyright: © 2024 by the author(s). Published by Maximum Academic Press on behalf of Hainan Yazhou Bay Seed Laboratory. This article is an open access article distributed under Creative Commons Attribution License (CC BY 4.0), visit https://creativecommons.org/licenses/by/4.0/.
-
About this article
Cite this article
Wang CY, Wang ML, Li QQ, Yan Y, Hao S, et al. 2024. Enhancing the antioxidant potential of wheatgrass to improve nutrient value. Seed Biology 3: e012 doi: 10.48130/seedbio-0024-0010
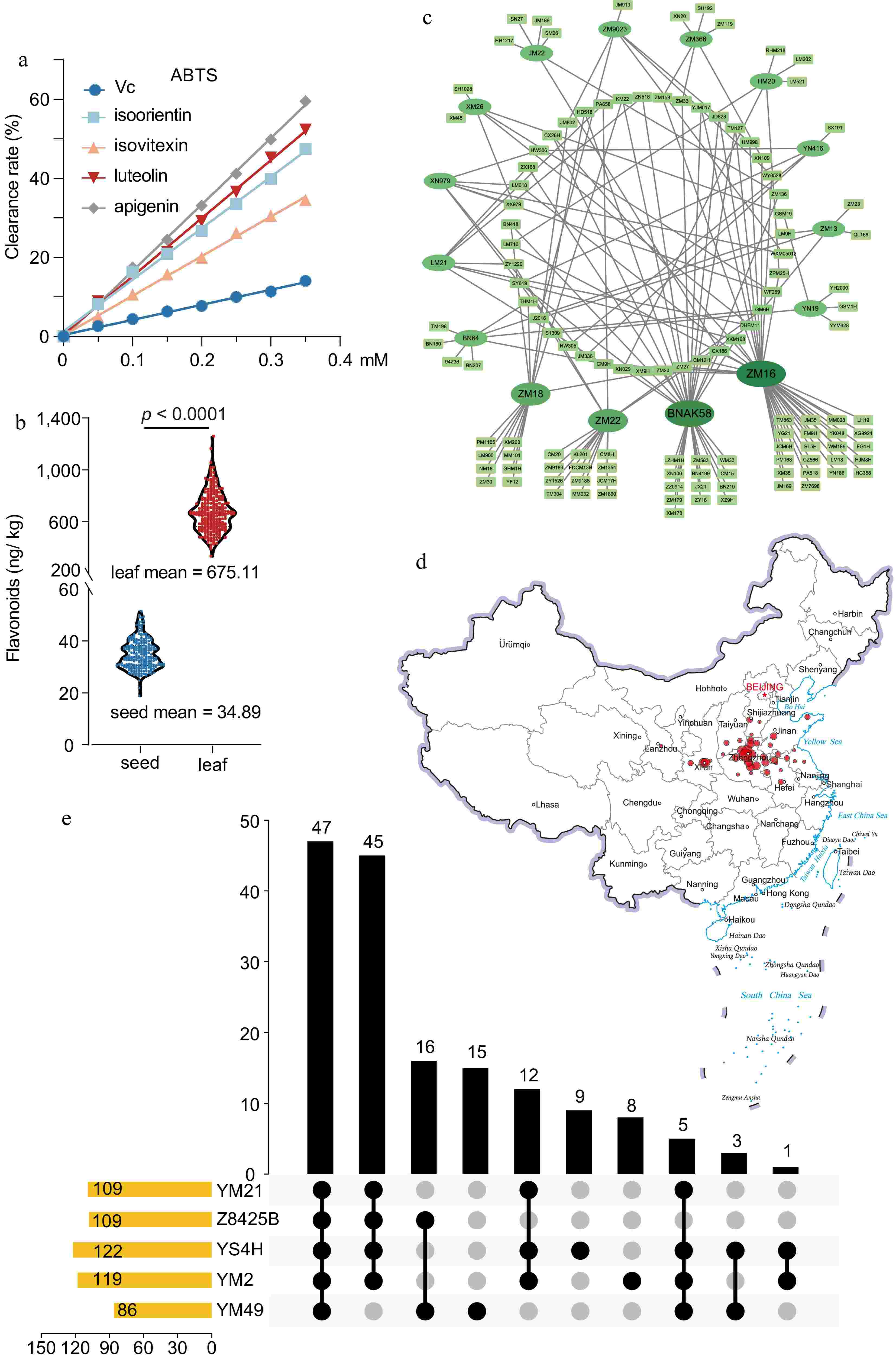