-
Drought is a primary environmental factor that adversely affects crop production. Over half of the worlds crops are cultivated in arid and semi-arid regions, where drought significantly reduces crop yield and quality[1]. Under water deficit conditions, the excessive accumulation of reactive oxidative species (ROS) can severely impair plant growth and development[2]. In response, plants have evolved numerous sophisticated mechanisms to counteract dehydration, including regulating growth and maturation, reducing plant height, promoting root development, and accelerating leaf senescence and flowering[3]. For example, to mitigate drought stress, plants accelerate leaf senescence and promote flowering by reallocating water and nutrition from older to younger leaves and reproduction issues[4]. Furthermore, plant hormones, flavonoids, and microbiomes regulate drought stress resilience[5]. During drought stress, abscisic acid (ABA) accumulation induces stomatal closure and leaf senescence through increased ROS levels[6]. Therefore, plants modulate diverse molecular and physiological pathways to improve resilience to drought stress.
Melatonin, also known as 5-methoxy-N-acetyltryptamine, was first discovered in the pineal gland of bovine[7] and subsequently found in plants as early as 1995[8,9]. This multifunctional molecule is involved in plant growth and development, including germination, root architecture, flowering time, leaf senescence, and fruit ripening[10]. Moreover, melatonin has emerged as a critical regulator of plant resilience to various biotic and abiotic stresses, including salinity, extreme temperatures, heavy metals, ultraviolet radiation, and drought[11]. Consequently, research investigating melatonin's functions and regulatory mechanisms in plants has expanded exponentially in recent years. Numerous studies indicated that melatonin alleviates drought stress by regulating various morphological, physiological, and biochemical processes, including seed germination, root and shoot development, leaf senescence, flowering, stomatal closure, photosynthetic efficiency, cell membrane integrity, osmotic stress, autophagy, etc[12]. For instance, seeds pretreated with melatonin had significantly improved germination under drought stress in various species, including hexaploid triticale (Triticale hexaploid L.), wheat (Triticum aestivum L.), and cotton (Gossypium hirsutum L.)[13,14]. Moreover, melatonin plays a positive role in root growth and a negative role in shoot development, facilitating water absorption and enabling plants to cope with drought stress.
These findings suggest the crucial role of melatonin on drought stress tolerance. However, comparing these studies is challenging due to the complexity of phenotypes and physiological activities under varying drought conditions. Factors such as drought treatment methods (osmotic stress or soil drought stress), duration of drought stress (hours or days), sampling positions (roots, stems, leaves, or whole plants), and culture conditions (greenhouse or field conditions) add to this complexity. Therefore, further studies are needed to explore the underlying mechanisms by which melatonin enhances drought resilience. In this review, the ROS-scavenging properties of melatonin and its metabolites are critically evaluated under drought stress conditions, alongside its regulation of growth, development, and physiological activities during drought conditions. Then, how melatonin enhances drought tolerance by regulating plant hormones, microorganisms, and flavonoids are discussed. In addition, several future research directions for melatonin are proposed that could advance agricultural production under drought conditions.
-
Melatonin biosynthesis in plants has been extensively studied following its discovery. Initially, researchers utilized heterologous expression of animal biosynthetic genes, such as hydroxyindole-O-methyltransferase (HIOMT) from sheep, to enhance plant melatonin levels[15]. Subsequent investigations revealed that melatonin can be biosynthesized in plants' chloroplasts and mitochondria[16,17]. In plants, both melatonin and indole-3-acetic acid (IAA) are synthesized from tryptophan. As shown in Fig. 1, melatonin biosynthesis involves two distinct pathways that involve six enzymes, including tryptophan decarboxylase (TDC), tryptamine 5-hydroxylase (T5H), serotonin N-acetyltransferase (SNAT), N-acetylserotonin methyltransferase (ASMT), and caffeic acid O-methyltransferase (COMT) (Fig. 1). Then, numerous studies have established that overexpression or knockout melatonin biosynthesis genes can directly modulate melatonin levels, thereby regulating plant stress tolerance[18−29] (Table 1). In Arabidopsis, overexpression of TaCOMT enhanced drought tolerance by promoting root growth and increasing proline content[30]. Therefore, further studies could use genetic modification techniques, such as CRISPR-Cas9, to improve plant drought tolerance by regulating melatonin biosynthesis genes in crops.
Figure 1.
Melatonin biosynthetic pathways in plants. Solid lines indicate enzymes or oxidants identified in plants, whereas dashed lines indicate enzymes or oxidants identified in animals but not yet confirmed in plants. AFMK: N1-acetyl-N2-formyl-5-methoxyknuramine, AMK: N-acetyl-5-methoxyknuramine, ASDAC: N-acetylserotonin deacetylase, ASMT: N-acetylserotonin methyltransferase, COMT: caffeic acid O-methyltransferase, IAA: indole-3-acetic acid, IDO: indoleamine 2,3-dioxygenase, IPA: indole-3-pyruvate, M2H: melatonin 2-hydroxylase, M3H: melatonin 3-hydroxylase, ROS: reactive oxygen species, RNS: reactive nitrogen species, SNAT: serotonin N-acetyltransferase, TAA: tryptophan aminotransferase of Arabidopsis, TDC: tryptophan decarboxylase, T5H: tryptamine 5-hydroxylase, TPH: tryptophan hydroxylase, YUC: YUCCA (the YUCCA gene encodes a flavin monooxygenase-like enzyme), 2-ODD: 2-oxoglutarate-dependent dioxygenase, 2-OHM: 2-hydroxymelatonin, 3-OHM: 3-hydroxymelatonin, 4-OHM: 4-hydroxymelatonin, 6-OHM: 6-hydroxymelatonin.
Table 1. Regulation of melatonin biosynthesis genes to enhance stress tolerance in plants.
Object species name Melatonin biosynthesis genes Function of melatonin Ref. Apple
(Malus domestica Borkh.)MdASMT9 Overexpression of MdASMT9 also reduces abscisic acid accumulation through promoting MdWRKY33-mediated transcriptional inhibition of MdNCED1 and MdNCED3, thus inducing stomatal opening for better heat dissipation. [18] Arabidopsis thaliana L. AtSNAT2 snat2 knockout mutant exhibits delayed flowering and reductions in leaf area and biomass. [19] Barley
(Hordeum vulgare L.)HvCOMT1, HvCOMT2, and HvCOMT3 Reducing thioacidolysis yields in the COMT RNAi lines are an indication of changes to lignin structure with a greater proportion of resistant bonds in the lignin. [20] Grapevine
(Vitis vinifera L.)VvASMT1 Ectopic overexpression of VvASMT1 in Nicotiana benthamiana significantly enhances melatonin production and increased tolerance to salt and osmotic stresses. [21] Malus zumi Mats. MzASMT1 Overexpression of MzASMT1 increases melatonin levels and drought tolerance in Arabidopsis. [22] Rice
(Oryza Sativa L.)OsASMT1, OsASMT2, and OsASMT3 All three ASMT mRNAs are simultaneously induced in treatments with abscisic and methyl jasmonic acids. [23] Rice
(Oryza Sativa L.)OsCOMT Overexpression of OsCOMT significantly delays leaf senescence at the grain-filling stage by inhibiting the degradation of chlorophyll and chloroplast. OsCOMT plays a positive role in the vascular development of rice. OsCOMT is a positive regulator of grain yield. [24] Rice
(Oryza Sativa L.)OsSNAT1 OsSNAT1-overexpressing rice plants increases resistance to cadmium and senescence stresses. [25] Rice
(Oryza Sativa L.)OsSNAT2 SNAT2 RNAi lines indicates a decrease in melatonin and a dwarf phenotype with erect leaves by exhibiting photomorphogenic phenotypes such as inhibition of internodes and increased expression of light-inducible CAB genes in the dark. [26] Tobacco
(Nicotiana tabacum L.)NtCOMT1 Overexpression of NtCOMT1 promotes drought resistance by increasing melatonin content. [27] Watermelon
(Citrullus lanatus L.)ClCOMT1 Overexpression of ClCOMT1 enhances transgenic Arabidopsis tolerance against cold, drought, and NaCl. [28] Tomato
(Solanum lycopersicum L.)SlCOMT1 Overexpression of COMT1 significantly enhances the capacity of the tomato to reduce fungicide carbendazim phytotoxicity and residue. [29] Wheat
(Triticum aestivum L.)TaCOMT Overexpression of the wheat TaCOMT gene enhances drought tolerance and increases the content of melatonin in transgenic Arabidopsis. [30] Cassava
(Manihot esculenta Crantz)MeTDC2, MeASMT2, and MeASMT3 MeWRKY20/75 interacts with 3 melatonin synthesis enzymes (MeTDC2, MeASMT2/3) and positively regulates endogenous melatonin accumulation. [55] Cassava
(Manihot esculenta Crantz)MeTDC2, MeT5H, MeASMT1, MeTDC1, MeASMT2, MeASMT3, and MeSNAT MeRAV1 and MeRAV2 positively regulate 7 melatonin biosynthesis genes (MeTDC2, MeT5H, MeASMT1, MeTDC1, MeASMT2, MeASMT3, and MeSNAT) and the endogenous melatonin level in plant disease resistance against cassava bacterial blight. [56] In addition, several studies demonstrated that high melatonin content enhances stress tolerance by increasing plant flavonoid biosynthesis[31]. On the contrary, some flavonoids, including morin and myricetin, can significantly decrease melatonin content by inhibiting the expression of SNAT1 and SNAT2 in rice (Oryza sativa L.)[32]. Only several studies indicate that heterogeneous overexpression of melatonin biosynthesis genes significantly enhances drought tolerance[33]. However, no studies have demonstrated the regulation of melatonin biosynthesis genes by transcription factors under drought conditions. Hence, further studies can focus on identifying the upstream transcription factors that interact with melatonin biosynthesis genes during drought stress.
Exogenous application of melatonin enhances drought tolerance
-
Exogenous application of melatonin has been widely used to increase melatonin levels within the plant to enhance stress tolerance. Indeed, appropriate concentrations of melatonin can bolster drought tolerance in various plant species, including apple (Malus domestica Borkh.)[34,35], alfalfa (Medicago sativa L.)[36], maize (Zea mays L.)[37], tomato (Solanum lycopersicum L.)[38−41], wheat[42,43], rice[33], cotton[44], etc. Exogenous melatonin is typically applied through foliar spraying, root application, or seed priming[45−52] (Table 2). However, most of these studies are investigating the application of melatonin in greenhouse conditions across various species. Hence, future studies should focus on developing melatonin-related biostimulants for sustainable agricultural production in field conditions. This may involve investigating optimal application methods, timing, dosage, and other relevant factors under drought conditions. Overall, one of the research directions is to incorporate melatonin and its metabolites into crop breeding programs to enhance food production and security.
Table 2. Exogenous melatonin enhances drought tolerance in plants.
Species name Melatonin concentration Function of exogenous melatonin under drought stress Ref. Apple
(Malus domestica Borkh.)Root application (100 μM) Helping to maintain the better function of PSII and controlling the burst of hydrogen peroxide to delay the leaf senescence under drought. [3] Cotton
(Gossypium hirsutum L.)Foliar spray (100 μM) Down-regulating chlorophyll degradation-related genes and senescence marker genes (GhNAC12 and GhWRKY27/71). Improving photosynthetic efficiency, reducing chlorophyll degradation and ROS accumulation, and inhibiting ABA synthesis, thereby delaying drought-induced leaf senescence in cotton. [4] Wheat
(Triticum aestivum L.)Root application (100 μM) Alleviating photosynthetic and cell membrane damage by maintaining low levels of hydrogen peroxide. [13] Rice
(Oryza Sativa L.)Seed priming (100 μM) Promoting the germination rate and improving the biomass of rice seed shoots and roots. Alleviating the oxidative damage of rice seeds caused by drought stress. [33] Alfalfa
(Medicago sativa L.)Foliar spray (100 μM) Boosting antioxidant enzyme activities, improving photosynthetic performance, and accumulating total soluble sugar and proline content. [36] Maize
(Zea mays L.)Foliar spray (100 μM), and
root application (50 μM)Reducing the reactive oxygen species burst and enhancing the photosynthetic activity. [37] Pepper
(Capsicum annuum L.)Foliar spray (100 μM) Reducing oxidative stress and improving nitrogen metabolism by activating various enzymes such as nitrate reductase, nitrite reductase, glutamine synthetase, and glutamine dehydrogenase activities. [38] Tomato
(Solanum lycopersicum L.)Seed priming (100 μM) Increasing stomatal conductance, photochemical efficiency, and the antioxidant system, and also reducing the cellular content of toxic substances. [39] Tomato
(Solanum lycopersicum L.)Foliar spray (100 μM), and
root application (100 μM)Alleviating the inhibition of drought stress on the gas exchange parameters and the leaf net photosynthetic rate, protecting the thylakoid membrane from damage, and strengthening the ATP-synthase activity. [40] Two Citrus cultivars Foliar spray (100 μM) Increasing total flavonoid and total phenolic contents under severe drought stress. [41] Wheat
(Triticum aestivum L.)Root application (500 μM) Decreasing membrane damage, more intact grana lamella of chloroplast, higher photosynthetic rate, and maximum efficiency of photosystem II, as well as higher cell turgor and water-holding capacity. [42] Wheat
(Triticum aestivum L.)Root application (100 μM) Improving fine root, lateral root, and root hair, plant height, dry weight, net photosynthesis, and stomatal aperture of leaves. [43] Cotton
(Gossypium hirsutum L.)Foliar spray (100 and 200 μM) Improving the translocation of carbon assimilates to drought-stressed anthers, regulating the carbohydrate balance of drought-stressed anthers to improve male fertility. [44] Kiwifruit
(Actinidia chinensis Planch)Root application (100 μM) Improving photosynthesis by inhibiting stomatal closure, enhancing light energy absorption, and promoting electron transport in PSII. [45] Maize (Zea mays L.) Foliar spray (100 μM) Increasing the accumulation of flavonoid metabolites, particularly apigenin, luteolin, and quercetin. Upregulating the expression of genes related to flavonoid biosynthesis (PAL, C4H, 4CL, HCT, CHS, CHI, F3′5′H, and DFR), activates drought-responsive transcription factors (ERFs, NACs, MYBs, and bHLHs). [46] Moldavian balm
(Dracocephalum moldavica)Foliar spray (100 μM) Increasing soluble sugar content, malondialdehyde content, and lipoxygenase activity, non-enzyme antioxidants (including flavonoid, polyphenol compounds, and anthocyanin) under moderate and severe drought stress. [47] Maize (Zea mays L.) Root application (100 μM) Improving the photosynthetic activities and alleviated the oxidative damages of maize seedlings under the drought stress. [48] Rapeseed
(Brassica napus L.)Root application (50 μM) Alleviating the seedling growth inhibition and increasing the leaf area and fresh and dry weights of roots and shoots under drought stress. [49] Rice
(Oryza Sativa L.)Root application (100 μM) Promoting root, shoot length, fresh and dry weight, and increasing chlorophyll contents. [50] Soybean
(Glycine max L.)Foliar spray or root application (50 and 100 μM) Improving photosynthetic activity, reduction of abscisic acid and drought-induced oxidative damage. [51] Tomato
(Solanum lycopersicum L.)Root application (150 μM) Affecting stomatal conductance and the activity of ROS scavenging enzymes. [52] Tomato
(Solanum lycopersicum L.)Root application
(100 and 500 μM)Carbon monoxide is a downstream signal molecule of melatonin-enhanced drought resistance by promoting chlorophyll biosynthesis. [59] Cotton
(Gossypium hirsutum L.)Seed priming (10 μM) Increasing photosynthetic activity, water-use efficiency, and nitrogen metabolism. Upregulating the expression of the autophagosome marker [lipidated (ATG8-PE)]. [63] Tomato
(Solanum lycopersicum L.)Root application (100 μM) Improving the seedlings growth, root characteristics, leaf photosynthesis and antioxidant machinery. [64] Creeping bentgrass
(Agrostis stolonifera)Foliar spray (20 μM) Increasing visual quality, photochemical efficiency, chlorophyll content, and relative water content. Up-regulating and chlorophyll-degradation genes, and cytokinin-signaling and synthesis genes. [82] Naked oats
(Avena nuda L.)Foliar spray (100 μM) Increasing the chlorophyll content and photosynthetic rate of leaves, increasing expression of PYL, PP2C, ABF, SNRK2, and IAA. [84] Barley
(Hordeum vulgare L.)Root application (2 mM) Exogenous melatonin increases the relative abundance of the bacterial community in carbohydrate and carboxylate degradation while decreasing the relative abundance in the pathways of fatty acid and lipid degradation and inorganic nutrient metabolism under drought. [89] -
Melatonin can stabilize membrane integrity by reducing ROS content under drought conditions. Previous studies demonstrated that melatonin plays a pivotal role in maintaining ROS homeostasis, regulating plant growth, and enhancing plant stress resilience[53]. On the one hand, melatonin reduces ROS levels by increasing antioxidant systems under stress conditions. On the other hand, melatonin also directly interacts with ROS/RNS to produce various metabolites, including 2-hydroxymelatonin (2-OHM), 3-hydroxymelatonin (3-OHM), 4-hydroxymelatonin (4-OHM), 6-hydroxymelatonin (6-OHM), 5-methoxytryptamine (5-MT), N1-acetyl-N2-formyl-5-methoxykynuramine (AFMK), and N-acetyl-5-methoxykynuramine (AMK) (Fig. 1). In addition, several plant enzymes involved in melatonin metabolism have been identified, including melatonin 2-hydroxylase (M2H), melatonin 3-hydroxylase (M3H), indoleamine 2,3-dioxygenase (IDO), and N-acetylserotonin deacetylase (ASDAC), which produce 2-OHM, 3-OHM, AFMK, and 5-MT, respectively. Further studies have indicated that these metabolites exhibit higher antioxidant activity than melatonin under stress conditions. For example, 2-OHM could mitigate cadmium stress in cucumber (Cucumis sativus L.) and increase drought stress tolerance by regulating the antioxidant system[54]. Additionally, various transcription factors and flavonoids directly regulate melatonin biosynthesis genes in cassava (Manihot esculenta Crantz.). For example, the transcription factor MeWRKY20/75 interacts with three melatonin biosynthesis enzymes (MeTDC2, MeASMT2/3) to enhance melatonin levels[55]. These authors also revealed that MeRAV1 and MeRAV2 positively regulate melatonin biosynthesis genes to improve disease resilience against cassava bacterial blight[56]. However, further studies are required to elucidate the function and the mechanisms by which these melatonin metabolites mitigate drought stress by decreasing ROS levels.
Studies on drought also indicate that transcription factors, such as DEHYDRATION-RESPONSIVE ELEMENT-BINDING PROTEIN (DREB), NAC, MYBs, bZIPs, and WRKYs, play an important role in plant resilience[57]. Melatonin has been shown to modulate various physiological processes and developmental growth to enhance plant stress tolerance by interacting synergistically with key phytohormones, such as ABA and gibberellic acid (GA)[58]. These interactions are often mediated through intricate signaling networks and transcription factors, such as NAC and MYB, which regulate gene expression in response to various environmental factors[59]. Additionally, several studies have reported that melatonin delays flowering time by stabilizing DELLA proteins or promoting the transcription of flowering locus C (FLC) to inhibit flowering locus T (FT)[60]. However, the regulatory mechanisms of melatonin on leaf senescence and flowering transition under drought conditions require further investigation. In addition, autophagy is activated to degrade and recycle drought-induced proteins, reducing their toxicity. Previous studies demonstrated that melatonin regulates autophagy by an ABA-independent pathway to enhance drought tolerance. For example, Wang et al. initially discovered that melatonin regulates autophagy to mitigate the accumulation of oxidized proteins during oxidant stress (methyl viologen)[61]. In wheat, rab-related proteins (RAB5C and RAB11A) related to autophagy activation were accumulated by applying melatonin during osmotic stress conditions[62]. Subsequently, studies revealed that melatonin enhances drought tolerance by upregulating ATG8 and ATG8-PE expression related to autophagosomes in cotton[63]. Hence, plants regulate ABA-dependent or independent pathways to improve drought stress tolerance.
Preliminary findings indicate that melatonin regulates stomatal conductance enhances drought tolerance by accumulating the osmolytes, and improves water use efficiency. This regulatory mechanism also underpins the observed increase in photosynthetic efficiency under drought conditions[64]. For example, melatonin can mitigate the reduction of stomatal conductance to enhance photosynthetic efficiency in various plants under drought conditions[65]. In addition, melatonin was also shown to prevent disruption of the photosystems within the thylakoids and the reduction of chlorophyll, rubisco protein, etc., under drought conditions[66]. For example, Ye et al. report that melatonin alleviates chlorophyll degradation and disruption of fluorescence parameters caused by drought stress[67]. Exogenous application of melatonin could improve photosynthetic efficiency and grain filling rate, thereby enhancing grain yield potential under drought stress conditions in soybean (Glycine max L.)[68] and maize[69]. Melatonin can also protect the photosynthetic electron transport in photosystems (PSI and PSII) and restore the fluorescence parameters to increase photosynthetic capacity[70]. However, the regulatory mechanism of melatonin on photosynthesis under drought stress conditions remains largely unknown.
In addition, identifying receptor proteins is vital for studying melatonin's regulation and signal pathways in plants. The first phyto-melatonin receptor1 (Cand2/PMTR1), a putative G protein-coupled melatonin receptor-like protein, was identified in Arabidopsis[71]. In the study by Wei et al., melatonin was shown to regulate stomatal closure by inducing ROS and Ca2+ signaling pathways or activating mitogen-activated protein kinase (MAPK) cascades in guard cells[71]. The melatonin receptor mutant, cand2, exhibited reduced osmotic stress tolerance with ROS accumulation and exogenous melatonin could not revert this osmotic stress phenotype in Arabidopsis[71]. Subsequently, homologs of PMTR1 have been identified in other plants. For instance, overexpressing ZmPMTR1 in Arabidopsis enhanced osmotic stress tolerance by reducing the rate of transpiration[72]. However, Lee & Back argued that PMTR1 is not a G protein-coupled melatonin receptor as, under stress conditions, melatonin-activated MAPK (MPK3/6) cascades without the need for PMTR1[73]. In addition, it is noteworthy that previous studies have identified at least two receptors (MT1 and MT2) in animals[74]. Therefore, further investigations are necessary to confirm the receptor function of PMTR1 or identify new melatonin receptors and elucidate its signal transduction pathways.
Additionally, MAPK cascades are essential signal transduction pathways that respond to various environmental stresses, such as drought, salt, and pathogens. Previous studies have demonstrated that melatonin activates MAPK signaling in response to plant pathogens[75]. In addition, melatonin regulated chloroplast protein quality control (CPQC), which is critical for starch synthesis and reducing DNA damage by activating the plant MAPK pathways[76]. Recent studies have further elucidated melatonin's role in MAPK pathways under stress conditions. For example, in apples, melatonin-activated MdMPK3 and MdMPK6 upregulate the melatonin biosynthesis gene, MdASMT7, by phosphorylating the transcription factor MdWRKY17[77]. Similarly, in Sour jujube (Ziziphus jujuba var. spinose), melatonin could enhance salt resilience by upregulating MKK3 in the MAPK signaling pathway[78]. Despite these findings, the specific mechanisms by which melatonin activates the MAPK cascade to alleviate drought stress in plants remain underexplored. Therefore, further studies are needed to elucidate MAPK cascade pathways regulating drought resilience.
-
Recent studies have shown that melatonin enhances drought tolerance by modulating other plant hormones, microbiome communities, and flavonoids. Plant hormones are vital for drought resilience in plants through their regulation of growth, development, and numerous physiological processes. In contrast, melatonin promotes stomatal reopening by decreasing ROS and ABA levels under drought conditions[79]. New studies have suggested that melatonin achieves this by downregulating ABA biosynthesis genes and upregulating ABA catabolism genes[80]. Other studies have demonstrated that melatonin can inhibit ABA transport from roots to shoots during drought stress[81]. Melatonin can synergistically interact with cytokinin (CTK) to increase drought tolerance by upregulating dehydration-responsive genes (JUB1 and DREB2A) and alleviate drought-induced leaf senescence by increasing endogenous CTK content[82]. Additionally, melatonin increased brassinosteroid (BR) content by upregulating biosynthesis genes, including CYP750A1, CYP707A5, CYP707A7, CYP87A3, and CYP90D2, thereby improving drought and cold tolerance[83]. The exogenous application of melatonin increased salicylic acid (SA), GA, and IAA under imposed water deficit conditions[84,85]. Jasmonic acid (JA) levels, which are increased by drought stress and contribute to drought tolerance, were positively regulated by melatonin during drought conditions[86]. Overall, these studies demonstrate that melatonin plays a central role by modulating these plant hormones and reducing ROS concentration caused by water deficit conditions (Table 3). Although these studies illustrated that melatonin can regulate plant growth, development, and physiological processes to enhance drought resilience by changing plant hormones' content, its interactions with other plant hormones under drought conditions remain largely unexplored. Therefore, further detailed omics studies are essential to identify the transcription factors and regulatory elements involved in this signaling cascade.
Table 3. Melatonin regulatory pathways under drought conditions.
Species name Regulation objects Function of melatonin under drought conditions Ref. Cotton
(Gossypium hirsutum L.)Decreased ABA Melatonin can effectively enhance the antioxidant enzyme system, improve photosynthetic efficiency, reduce chlorophyll degradation and ROS accumulation, and inhibit ABA synthesis, thereby delaying drought-induced leaf senescence in cotton. [4] Wheat
(Triticum aestivum L.)Increased JA Up-regulating jasmonic acid biosynthesis genes and increasing jasmonic acid contents to mitigate drought stress. [13] Maize (Zea mays L.) Increased GA and IAA; decreased ABA Melatonin increases GA and IAA while reducing the ABA levels in leaves under drought conditions. [69] Apple
(Malus domestica Borkh.)Decreased ABA Melatonin scavenges H2O2 and reduces ABA by upregulating MdNCED3 and downregulating MdCYP707A1 and MdCYP707A2 to re-open stomatal under drought conditions. [79] Maize (Zea mays L.) Reduced ABA Reducing ABA accumulation and inducing stomatal reopening by inhibiting up-regulation of NCED1, and up-regulating ABA catabolic genes ABA8ox1 and ABA8ox3. [80] Soybean (Glycine max L.) Decreased ABA in leaves and increased ABA in roots Melatonin-received plant leaves accumulates less ABA but roots content higher ABA. Melatonin significantly suppresses ABA biosynthesis and signaling gene expression in soybean exposed to drought stress. [81] Creeping bentgrass
(Agrostis stolonifera)Increased CTK Melatonin synergistically interacts with cytokinins to suppress drought-induced leaf senescence. Increasing endogenous cytokinins content and upregulating cytokinins signal transduction genes and transcription factors. [82] Perennial ryegrass
(Lolium perenne L.)Increased BR Brassinosteroid plays a critical role in the melatonin-mediated mitigation of cold and drought stress by triggering antioxidant activities as well as enhancing the photosynthetic capacities. Brassinosteroid biosynthesis and it signaling pathway are induced by melatonin. [83] Barley
(Hordeum vulgare L.)Increased SA, GA, CK, and IAA; decreased ABA Melatonin increases levels of SA, GA, CK, and IAA, as well as a decrease in abscisic acid to enhance stress tolerance in barley. [84] Cotton
(Gossypium hirsutum L.)Increased GA and reduced ABA Increasing the germination rate, germination potential, radical length, and fresh weight, as well as the activities of superoxide dismutase (SOD), peroxidase (POD), and α-amylase. Melatonin alleviates drought stress by reducing ABA content and increasing GA3 content. [85] Maize (Zea mays L.) Increased JA Up-regulating jasmonic acid biosynthesis genes and increasing jasmonic acid contents to mitigate drought stress. [86] Melatonin regulates microbes to enhance drought stress
-
Plants can manipulate and recruit beneficial microbial communities to produce various compounds, including phytohormones, osmolytes, antioxidants, and volatile organic compounds (VOCs), that mediate in alleviating drought stress[87]. Under drought conditions, plant microbiomes also influence the expression of drought-related genes. For instance, a B. subtilis strain can induce the expression of drought-related genes, such as RD29 and DREB2, to mitigate drought stress in rapeseed (Brassica napus L.) and Arabidopsis[88]. Melatonin can also interact, synergistically, with arbuscular mycorrhizal fungi (AMF) to improve drought tolerance. Applying melatonin to roots can alter the composition of rhizosphere microbiomes, promoting bacteria involved in carbohydrate and carboxylate degradation, which was shown to help alleviate drought stress in barley[89]. In addition, melatonin affects the structure of microbial communities, including soil fungi and bacteria, leading to improved soil nitrogen content and utilization efficiency, thereby enhancing drought resilience[90]. Currently, the mechanism(s) by which melatonin interacts with the plant microbes to mitigate drought stress remains largely unknown. This is the new future research direction for improving plant drought tolerance. Future research should focus on elucidating the communication mechanisms between melatonin and microbial communities to mitigate drought stress in plants. Identifying specific bacterial or fungal communities that enhance melatonin's function in improving drought resilience represents a promising direction for the enhancement of global agriculture.
Melatonin regulates flavonoids to enhance drought tolerance
-
Flavonoids, such as anthocyanins, kaempferol, and quercetin, are specialized secondary metabolites known for their roles as phytoalexins or antioxidants, through their ROS-scavenging trait. Such flavonoids are crucial for protecting plants against both biotic and abiotic stress, including drought, as their accumulation has been demonstrated to significantly enhance drought tolerance. In addition, both melatonin and flavonoids have multifunctional roles in the plant and interact with plant microbes to regulate drought tolerance, as previously established.
Metabolomics analyses have shown that melatonin can regulate a variety of metabolites in the flavonoid biosynthesis pathway during drought stress conditions. For example, melatonin improves tolerance to salt, drought, and heat stresses, by upregulating the luteolin biosynthesis genes[91]. It also enhances stem strength by increasing lignin content and secondary cell wall thickness in herbaceous peonies[92]. Here, in particular, melatonin can increase the accumulation of apigenin, luteolin, and quercetin, under drought-stress conditions. In tobacco (Nicotiana tabacum L.), kaempferol, a type of flavonol, has also been reported to interfere with melatonin's regulation of ROS and stomatal closure[93]. Therefore, these recent studies confirmed that melatonin accumulates several different flavonoids to enhance drought tolerance. However, further studies are warranted to explore the relationship between melatonin and flavonoids in regulating drought tolerance. Especially the lack of molecular mechanisms between melatonin and different flavonoids under drought conditions.
-
In conclusion, current evidence indicates that melatonin, whether through the regulation of its biosynthesis genes or exogenous application, primarily functions by scavenging ROS. This activity helps to maintain ROS homeostasis and alleviates drought-induced stress. This pivotal role protects plants from drought-induced damage, including compromised seed germination, inhibited root growth, stomatal closure, decreased photosynthesis, etc. Moreover, melatonin mediates drought tolerance through intricate signal transduction pathways, potentially interacting with membrane receptors such as PMTR1 or activating mitogen-activated protein kinases (MAPKs) like MdMPK3 and MdMPK6. Additionally, recent investigations have elucidated melatonin's interactions with various phytohormones, plant microbes, and flavonoids, which further augment its role in drought tolerance (Fig. 2). For instance, melatonin synergizes with AMF to improve drought tolerance and modulates microbial community structures to enhance nutrient uptake and utilization. Metabolomics analyses have revealed that melatonin regulates differentially expressed metabolites in flavonoid biosynthesis, during drought stress, increasing the accumulation of key flavonoid metabolites, such as apigenin, luteolin, and quercetin.
Figure 2.
Schematic illustrating plant growth, development, and physiological activities regulated by melatonin under drought conditions. ABA: abscisic acid, AFMK: N1-acetyl-N2-formyl-5-methoxyknuramine, APX: ascorbate peroxidase, BR: brassinosteroids, CAT: catalase, CTK: cytokinin, DREB: dehydration-responsive element-binding protein, GA: gibberellic acid, GPA: G protein alpha subunit, GPX: glutathione peroxidase, IAA: indole-3-acetic acid, JA: jasmonic acid, MAPK: mitogen-activated protein kinase, MKK: MAPK kinase, MAPKKK: MKK kinase, PMTR1: phyto-melatonin receptor 1, RNS: reactive nitrogen species, ROS: reactive oxygen species, SA: salicylic acid, SOD: superoxide dismutase, TFs: transcription factors, 2-OHM: 2-hydroxymelatonin, 3-OHM: 3-hydroxymelatonin, 5-MT: 5-methoxytryptamine.
Despite these advancements, the mechanisms through which melatonin interacts with these various pathways to confer drought tolerance remains to be fully elucidated. Future research programs should focus on characterizing the detailed molecular and biochemical pathways involved in melatonin-mediated drought resilience. Identifying transcription factors and regulatory elements that interact with melatonin, as well as the role of melatonin in modulating plant microbiomes and other phytohormones are promising avenues for exploration. Hence, advanced omics approaches, such as transcriptomics, proteomics, and metabolomics will be instrumental in uncovering these complex interactions.
In summary, melatonin is a multifaceted molecule with significant potential in enhancing plant drought tolerance. By deepening our understanding of its underlying mechanisms, we can better harness melatonin's capabilities to mitigate drought stress in agricultural settings, thereby improving crop resilience and productivity in the face of climate change.
This work was supported by the China Scholarship Council (Contract No. 202003250115).
-
The authors confirm contribution to the paper as follows: study conception and design: Luo M, Chen M; data collection: Luo M, Zhang D, Tang W; analysis and interpretation of results: Delaplace P, Ma Y; draft manuscript preparation: Luo M, Zhang D. All authors reviewed the results and approved the final version of the manuscript.
-
Data sharing not applicable to this article as no datasets were generated or analyzed during the current study.
-
The authors declare that they have no conflict of interest.
-
Received 28 October 2024; Accepted 5 December 2024; Published online 14 February 2025
-
# Authors contributed equally: Mingzhao Luo, Dandan Zhang, Wensi Tang
- Copyright: © 2025 by the author(s). Published by Maximum Academic Press on behalf of Hainan University. This article is an open access article distributed under Creative Commons Attribution License (CC BY 4.0), visit https://creativecommons.org/licenses/by/4.0/.
-
About this article
Cite this article
Luo M, Zhang D, Tang W, Delaplace P, Chen M, et al. 2025. Recent advances in melatonin regulation of drought tolerance in plants. Tropical Plants 4: e005 doi: 10.48130/tp-0024-0047
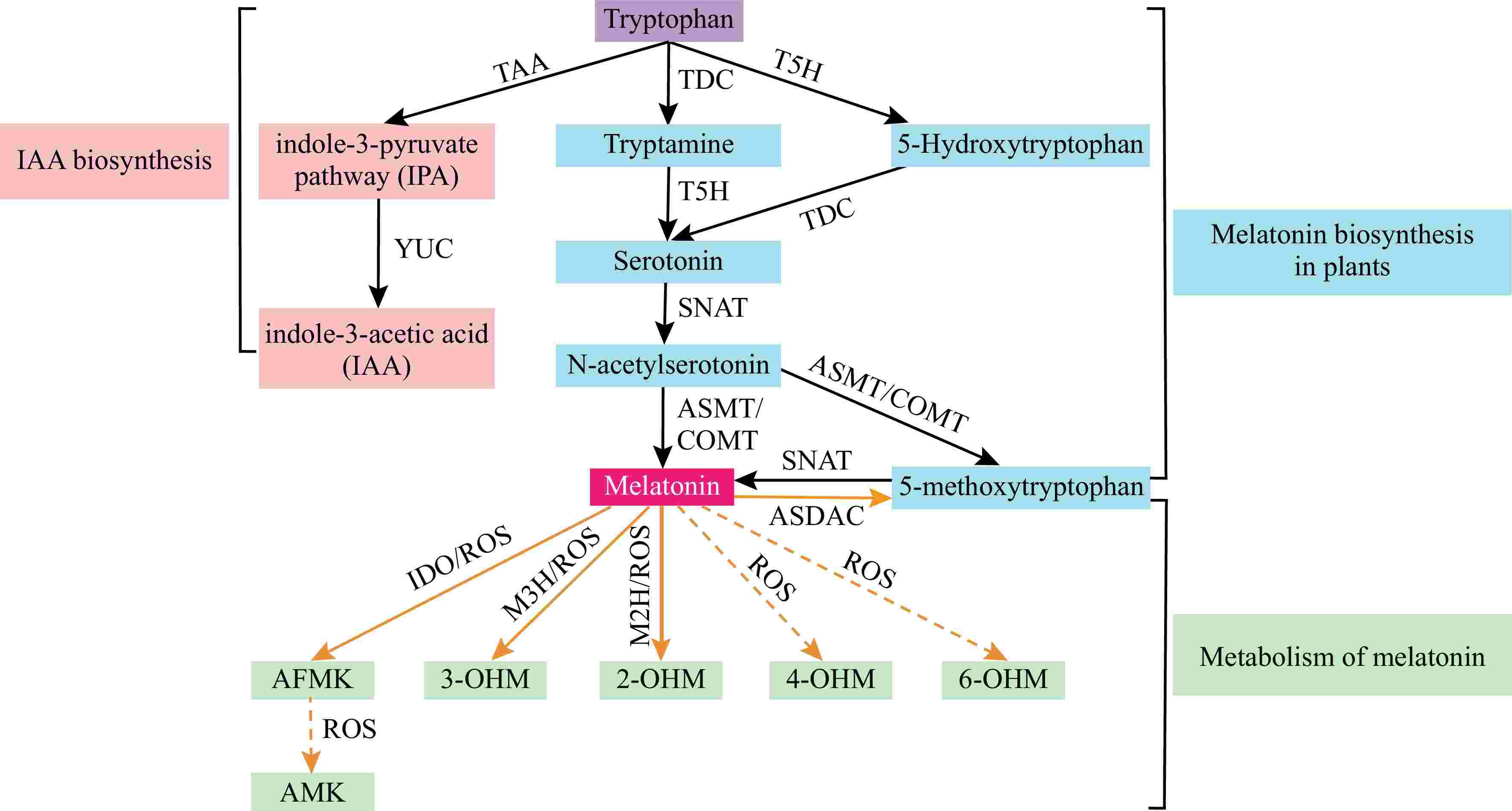