-
Aging entails the irreversible progression of physiological dysfunction and the onset of associated diseases within the living organism as its biological functions gradually decline over time[1]. Among the primary external contributors to aging are factors like radiation, food composition, and the environment in which one lives[2−5]. Conversely, intrinsic determinants predominantly encompass an excessive generation of free radicals, genomic instability, the shortening of telomeres, imbalances in protein homeostasis, anomalous proliferation and differentiation of stem cells, as well as insufficiencies in nutrient absorption[6]. Excessive levels of dynamic free radicals, such as reactive nitrogen species (RNS) and reactive oxygen species (ROS), can cause damage to cellular structures and metabolic processes. This damage often culminates in inflammation and oxidative stress, thus accelerating the aging process[7−10]. Wrinkles are a prominent characteristic of human skin aging which undergoes degeneration of collagen during the aging process. Free radicals decrease the biosynthesis of collagen and the proliferation of keratinocytes, and fibroblasts leading to a loosening of the connection between the epidermis and dermis layers of the skin. Therefore, depression of collagen synthesis and an increase in cell apoptosis are closely linked to the aging process[11]. Additionally, such factors collectively precipitate an array of aging-associated conditions like neurodegenerative disorders, skin aging, and metabolic syndrome, orchestrated through the aberrant mediation of cellular signaling pathways[12−15]. Given the substantial economic and psychological burdens borne by individuals afflicted with age-related ailments, the quest to avert or ameliorate aging's impact has garnered widespread attention The above factors would trigger the slew of aging-related diseases such as neurodegenerative diseases, skin aging, and metabolic syndrome through abnormal mediation of cell signaling transduction[16,17]. The challenge of preempting and alleviating diseases stemming from human aging thus emerges as a pivotal undertaking.
Compelling evidence underscores the paramount role of dietary restriction and nutrition in shaping health span and the trajectory of human aging[18,19]. Bioactive phytochemicals and nutrients inherent in our diet, including polyphenols, flavonoids, carotenoids, and bioactive peptides, exhibit a remarkable capacity to neutralize free radicals, thereby diminishing the likelihood of ROS and RNS. This process contributes to the extension of lifespan and the attenuation of aging markers within the organism. The objective of this review is to consolidate insights concerning the sourcing, isolation, and characterization of food-derived peptides in their role in countering aging-related maladies. We aim to explore the intricate interplay between molecular structure and the bioactive attributes of these concise peptides. Additionally, we endeavor to shed light on the potential mechanisms through which food-derived peptides operate, both in vivo and in vitro, to mitigate aging biomarkers. In essence, this succinct review endeavors to furnish guidance for the strategic application of food-derived peptides against aging-associated afflictions.
-
Animal-derived peptides with anti-aging properties encompass an array of sources, notably including whey peptides and collagen peptides extracted from diverse origins like eggs, fish, cattle, and pigs. Additionally, aquatic organisms such as sea cucumbers, mussels, locusts, and silkworms contribute to this repository[20−26] (Table 1). In 2010, Pei et al. succeeded in isolating a marine collagen peptide (MCP) of diminutive molecular weight—less than 0.86 kDa—from the skin of Oncorhynchus keta. This particular peptide was characterized by its abundance of polar amino acids[27]. Likewise, De Simone et al., in their endeavor to unlock the potential of buffalo milk waste whey (BWW), gleaned peptides with molecular weights ranging from 0.7 to 1.2 kDa through the processing of buffalo cheese whey[28]. Following this, in 2017, Song et al. achieved an impressive yield–50%–of peptides with molecular weights below 0.5 kDa, and a remarkable 70% of collagen peptides (CP) under 1 kDa via enzymatic hydrolysis of bovine bone collagen[22]. Adding to the intrigue, Qiu et al. embarked on a unique journey by synergizing Royal jelly and enzymatic hydrolysate from the skin of Carcharhinus falciformis, thereby obtaining a hybrid peptide—Royal jelly-collagen peptide (ERJ-CP)—wherein 95.8% of peptide molecules were under 1 kDa in weight[29].
Table 1. Summary of source, extraction, isolation, and identification of natural food-derived anti-aging peptides.
Species Sources Peptides Amino acid composition Molecular weight Extraction preparation Separation and purification Identification References Fungus Isaria japonica Naturido (DOPA)VLE 566.3 Da Solvent extraction (Water) RP-FCC, RP-HILIC-HPLC NMR, MS [41] Pyropia yezoensis PYP None None Enzymatic hydrolysis (Trypsin) CS MS [40] Pleurotus abalonus PPC None None Solvent extraction (Water) CS, GFC None [39] Plant Soybean WPK; AYLH WPK; AYLH 334.9 Da; 376.9 Da Enzymatic hydrolysis (Alkaline protease, Flavorzyme) AEC, GFC LC-MS/MS [75] Pea (Pisum sativum) Pep_RTE626 None 1,819.1 Da None None LC-MS/MS [37] Cardamine violifolia CSP None < 8 kDa Enzymatic hydrolysis (Alkaline protease, Neutral protease) CF None [33] Rice bran KF-8 KHNRGDEF 1,002.6 Da None HPLC HPLC, MS [34] Chia seed (Salvia hispanica L.) CSPs None < 3 kDa Enzymatic hydrolysis (Alkaline protease, Flavourzyme) UVAF HPLC, LC-MS/MS [35] Lotus japonicus somatic embryo LjSEC-EP PDLGSAVTRFIYKCMHEC(Taurine) < 0.8 kDa Enzymatic hydrolysis (Protease) CF MS, NMR, AAA [38] Rapeseed meal RP-1 EPLVKFGDIRTHMSYC < 3 kDa Fermentation (Bacillus subtilis) CF, UF, IEC None [54] Potato (Solanum tuberosum L.) APPH None 5% > 6 kDa, 95% < 6 kDa Enzymatic hydrolysis (Alkaline protease) UF, RP-HPLC MS/MS [31] Potato PHP None None Fermentation (Saccharomyces cerevisiae) None None [53] Angelica sinensis AsiPeps None < 3 kDa Enzymatic hydrolysis (Trypsin, Papain) CF, UF, GFC LC-MS/MS [32] Black soybean (Glycine max) BSPs GSTHDERACMKVLPFYI None Enzymatic hydrolysis (Alkaline protease, Neutrase, Flavourzyme) UF, MARC AAA [30] Animal Whey WHP EDLKTIPVASFYRCMWGH(Hyp) None Enzymatic hydrolysis None None [26] Whey WHP None None None None None [83] Whey WHP None 356 Da None None None [75] Whey BWW None < 1.2 kDa None CF, UF,
RP-HPLCMS/MS [28] Collagen PCP None None None None None [25] Collagen CP; EP KWFMTILVHDEGAYRPCS; KWFMTILVHDEGAYRPCS None None None AAA [62] Collagen CP DESGHTAPRYVMCILFK 70% < 1 kDa Enzymatic hydrolysis (Alkaline protease) None HPLC, RP-HPLC [22] Gadus morhua SWPI; SWPII DECSGHRTAPYVMWILFK 4976 Da; 1,960 Da Enzymatic hydrolysis (Protamex) VC, GFC FT-IR, UV, RP-HPLC [42] Crimson snapper CSSPs DESHGTRAYCVMFILKP 99% < 3 kDa Enzymatic hydrolysis (Papain) None AAA, HPLC [43] Fish FHS None < 1 kDa None None None [67] Fish CPNS GP; P(Hyp) 800 Da Enzymatic hydrolysis (Multiple fungal proteases) HPLC GPC, LC-MS/MS [23] Oncorhynchus keta MCP GEP(Hyp)DARKLSVITFHMY < 0.86 kDa Enzymatic hydrolysis (Trypsin, Papain, Alkaline proteinase) RP-HPLC RP-HPLC, MS, AAA [27] Apostichopus japonicus AJPH None < 3 kDa Enzymatic hydrolysis (Typsin, Papain) UF, GFC RP-LC-MS/MS [47] Stichopus variegates SVPF None < 3 kDa Enzymatic hydrolysis (Protamex) UF UPLC-MS/MS [48] Royal jelly and Carcharhinus falciformis ERJ-CP GEDAPRKYLSVMLFHTC 95.8% < 1 kDa Enzymatic hydrolysis (Alkaline protease, Flavourzyme) CF AAA, HPLC [29] Locusta migratoria manilensis LP-1 TFKHG 589.3 Da Solvent extraction (Isopropyl alcohol) UF, HC,
AEC, GFC,
RP-HPLCAAA, LC-MS [24] Mytilus edulis MP None None None None None [21] Bombyx mori SP-NN; SP-PN None 962.9 Da Enzymatic hydrolysis (Neutrase, Protease N, Protease P) None None [20] The abbreviations in Table 1 are all listed in Supplemental Tables S1 & S2. Anti-aging peptides derived from plant sources within natural foods primarily originate from a diverse range of plants, including soybean, black soybean, Pisum sativum, Cardamine violifolia, chia seed, Angelica sinensis, potato, and rice bran[30−37] (Table 1). In a noteworthy example, Wang et al. harnessed enzymatic hydrolysis to extract Angelica sinensis peptides (AsiPeps) with molecular weights under 3 kDa. This formulation exhibited no dietary restrictions upon Caenorhabditis elegans[32]. In the year 2019, Tito et al. employed enzymatic hydrolysis to derive peptides from Lotus japonicus somatic embryos (LjSEC-EP), with molecular masses spanning from 0.4 to 0.8 kDa, featuring a hydrophobic N-terminal[38]. In parallel, Wang et al. isolated a rice bran-derived bioactive peptide (KF-8) boasting eight amino acids (1,002.6 Da) and a substantial content of polar amino acids[34]. Another intriguing finding by Yu et al. encompassed selenium-enriched peptides from Cardamine violifolia (CSP), characterized by molecular weights below 8 kDa[26]. Moreover, Amakye et al. uncovered Trp-Pro-Lys (WPK, 334.9 Da) and Ala-Tyr-Leu-His (AYLH, 376.9 Da) within soybeans. Notably, these peptides exhibited aromatic amino acids and hydrophobic N-terminal properties[36].
Microorganisms serve as prominent sources of natural food-derived anti-aging peptides, with Pleurotus abalonus, Pyropia yezoensis, and Isaria japonica occupying the forefront of this domain[39−41] (Table 1). Li et al. adopting water as a solvent, orchestrated the extraction of a polysaccharide–peptide complex (PPC) from Pleurotus abalonus. This intricate structure consisted of carbohydrates and proteins, constituting 45.79% and 13.86% of Pleurotus abalonus, respectively[39]. Oh et al. harnessed enzymatic hydrolysis to procure phycoerythrin-derived tryptic peptide from Pyropia yezoensis (PYP). This peptide exhibited potent neural anti-aging attributes[40]. Recent research by Ishiguro et al. unveiled Naturido, a peptide containing merely 4 amino acids and weighing 566.3 Da, isolated from Isaria japonica through solvent extraction[41]. Given the wealth of food-derived peptides, ongoing research endeavors will invariably center around the investigation of marine fish-derived peptides and fungi exhibiting anti-aging properties[22,25,36,39−42]. Furthermore, there has been a growing research interest in harnessing marine resources and uncovering peptides with anti-aging properties. A compendium of evidence underscores that both marine organisms and fungi hold immense potential as a repository for identifying novel peptides endowed with anti-aging attributes[40−43]. The exploration of bioactive proteins or peptides sourced from marine fish not only unveils compounds advantageous for human well-being but also underscores the potential utility of by-product marine resources—an environmentally conscious strategy poised to play a pivotal role in the future
Structural characteristics and properties of peptides
-
Comprehensive analysis of the amino acid composition with existing natural food-derived anti-aging peptides underscores the following peptide characteristics: (1) 'Compact Size': Peptides with concise amino acid sequences (less than 20 amino acids) display heightened absorption efficiency, enabling them to traverse the intestinal barrier while preserving their in vivo biological activity; (2) 'Hydrophobic N-Terminal': The presence of a hydrophobic N-terminal within peptides facilitates hydrogen donor interactions with other amino acids, thereby augmenting their anti-aging potency; (3) 'Abundance of Polar Amino Acids': A substantial proportion of polar amino acids confers the ability to thwart free radical oxidation through the chelation of polar amino acid side chains; (4) 'Aromatic Amino Acid Inclusion': The peptide structure's incorporation of aromatic amino acids is pivotal. These aromatic residues readily release protons to electron-deficient free radicals, culminating in robust anti-aging activity[29,30,38,43]. Previous studies have shown the antioxidative potential of specific amino acids such as hydroxyproline (Hyp), leucine (Leu), alanine (Ala), and valine (Val) establishing their viability for application as antioxidants[44] (see Fig. 1).
Extraction and preparation of natural food-derived anti-aging peptides
Enzymatic extractions
-
The fundamental concept underlying the production of peptides through enzymatic hydrolysis revolves around the utilization of multiple enzymes featuring diverse cleavage sites. These enzymes act on an array of protein substrates, resulting in the formation of polypeptide fragments and amino acids[45]. This methodology is currently the main method used for extracting anti-aging peptides and offers distinct advantages, including manageable and moderate reaction conditions that foster the retention of peptide biological activity, alongside minimal chemical residue formation. However, this approach does entail certain drawbacks, notably elevated costs, protracted reaction durations, enzyme variability, and the challenge of precise reaction control[46]. Several primary categories of enzymes contribute to this process, including alkaline protease, neutral protease, flavourzyme, trypsin, papain, and protamex[33,36,42,47] (Table 1). In specific instances, Chiang et al. & Song et al. used alkaline protease to prepare alcalase treatment-derived potato protein hydrolysate (APPH) and collagen peptides from Olanum tuberosum L. and bovine bone, separately[22,31]. Additionally, Chen et al. employed papain to craft collagen and selenium-enriched peptides from Crimson snapper[43].
However, in most cases, the synthesis of the desired peptide mandates the harmonious interplay of multiple enzyme types. Wang et al. & Guo et al. for instance, achieved triumph in crafting AsiPeps and AJPH, hailing from Angelica sinensis and Apostichopus japonicus, respectively, by ingeniously merging typsin and papain[32,47]. Similarly, two separate studies disclosed the assembly of SWP I/II and Stichopus variegates peptide fraction (SVPF) through the composite protease protamex. The former materialized from the swim bladder of Gadus morhua, while the latter emerged from Stichopus variegates[42,48]. Aguilar-Toalá et al. & Amakye et al. used chia seed and soybean as substrates to prepare chia seed peptides (CSPs) and WPK/AYLH, individually, via combining alkaline protease with flavorzyme[35,36]. It's worth noting that certain naturally occurring peptides with senescence-related attributes mandate enzymatic digestion through specialized proteases. Park et al. in 2011, harmonized neutral protease, amino G protease from Bacillus subtilis, and amino 6 G protease from Aspergillus melleus to fabricate silk peptide-NN (SP-NN) and silk peptide-PN (SP-PN), utilizing silk as the substrate[20]. Subsequently, Lee et al. prepared collagen peptide NS (CPNS) using a range of fungal proteolytic enzymes[23].
Solvent extractions
-
The principle of solvent extraction was that the cleavage of peptide bonds is formed by employing acidic or alkaline reagents[49]. Among the prevalent solvents employed to extract anti-aging peptides from natural food sources, water, and isopropanol stand out as the primary choices[24,39,41] (Table 1). The solvent-based extraction approach offers the advantages of simplicity, cost-effectiveness, and user-friendliness, rendering it readily accessible and manageable. Nonetheless, this method does have its limitations. Notably, water-based peptide extraction exhibits sub-optimal efficiency. Furthermore, the application of acid and alkali for extraction carries the inherent risk of inducing protein denaturation and subsequent inactivation, thus compromising safety considerations[50].
Fermentation extractions
-
The fermentation of food represents a method for disintegrating active peptide fragments or amino acids from proteins through the action of diverse proteases produced by bacteria[51]. The benefits inherent to the fermentation approach encompass cost-effectiveness, a straightforward procedural framework, and heightened utilization of raw materials. Furthermore, the microbial fermentation process yields a spectrum of proteases, effectively circumventing the predicament of incomplete hydrolysis attributable to a sole enzyme[52]. However, the disadvantage of this method is that the demand for strains is great, the duration of fermentation is long, and the selectivity is low[49]. In the domain of preparing natural food-derived anti-aging peptides through fermentation, the pivotal strains employed include Bacillus subtilis and Saccharomyces cerevisiae[53,54] (Table 1).
Traditional methods for extracting peptides from food sources include enzymatic hydrolysis, solvent extraction, and fermentation. However, these methods yield functional peptides in very low quantities, severely restricting their potential for further exploration and utilization in the food and pharmaceutical industries. To address this limitation, the following strategies could be devised: (1) Enhancing the efficiency of enzymatic hydrolysis: Consider optimizing high-efficiency enzymatic hydrolysis of peptides, possibly through the synergistic action of novel enzymes or fermentation techniques[55]. (2) Employing advanced recombinant expression systems for peptide biosynthesis: The creation of peptides with optimal activities can be accomplished using cutting-edge recombinant expression systems. This approach eliminates the need for intricate isolation and purification procedures, expediting the industrial application of specific pharmaceutical peptides[56,57]. (3) Chemical synthesis of bioactive peptides: Bioactive peptides with anti-aging properties can be efficiently synthesized through chemical methods based on amino acid units[58,59]. This ongoing technique continues to propel the pharmaceutical utilization of bioactive peptides. In future, the evolution of techniques for isolating and producing bioactive peptides from food sources will play a pivotal role in significantly enhancing the industrial processing of natural food-derived anti-aging peptides.
Isolation, purification, and identification of natural food-derived anti-aging peptides
Isolation and purification
-
The purification process of natural food-derived anti-aging peptides typically encompasses various techniques such as chromatography, reversed-phase high-performance liquid chromatography (RP-HPLC), gel filtration chromatography (GFC), anion exchange chromatography (AEC), and macroporous adsorption resin chromatography (MARC), along with supplementary methods like ultrafiltration and centrifugation[24,30,36,41,54] (Table 1). Depending on the molecular weight, peptides can be separated and refined through either centrifugation or ultrafiltration. Notably, recent investigations have successfully achieved the isolation of ERJ-CP, LjSEC-EP, and CSP (< 1 KDa) through individual centrifugation methodologies[29,33,38]. It is worth mentioning that Lin et al. separated and purified peptide SVPF using ultrafiltration separation and acid heat treatment to effectively degrade sea cucumber peptides, and maintain their biological activities[48].
The application of chromatography, often involving multiple techniques, in the separation and purification of anti-aging peptides is founded on the principle that diverse substances possess distinct partition coefficients within a system comprising a stationary phase and a mobile phase. Consequently, they exhibit disparate retention times during elution with the mobile phase, thus achieving effective separation[60]. Several notable instances underscore the efficacy of this approach. Pei et al.[27], Lee et al.[23] and Liang et al.[61] successfully purified MCP, CPNS, and KF-8 by RP-HPLC respectively. Amakye et al. not only achieved the initial purification of WPK and AYLH peptides via AEC but also further separated and purified these peptides employing GFC[36]. A synergistic combination of ultrafiltration, centrifugation, and chromatography is frequently harnessed to segregate and refine anti-aging peptides. De Simone et al. purified peptide BWW by centrifugation, ultrafiltration, and RP-HPLC[28]. Then, Guo et al.[47] and Li et al.[42] separated and purified AJPH and BSPs, independently, by ultrafiltration combined with GFC and MARC. Cao et al. were able to extract the purified peptide LP-1 using hydrophobic chromatography, ultrafiltration fractionation, AEC, GFC, and RP-HPLC[24].
In conclusion, various methods are available for separating food-derived anti-aging peptides based on their physical and chemical properties, such as molecular weight and polarity. For example, RP-HPLC separates hydrophobic peptides, while ion exchange chromatography is suitable for charged peptides. Centrifugation or ultrafiltration can be used for peptides with specific molecular weights. Researchers are increasingly combining multiple chromatographic techniques with ultrafiltration and centrifugation.
Identification
-
The predominant method employed for elucidating the structure of natural food-derived anti-aging peptides involves the utilization of an amino acid analyzer (AAA)[30,38,62] (summarized in Table 1). Pei et al.[27], Qiu et al.[29] and Cao et al.[24] used the H835-50 automatic amino acid analyzer, high-speed amino acid analyzer Model L-8900, and Hitachi high-speed amino acid analyzer 835-50, respectively, to detect the amino acid composition of peptides MCP, ERJ-CP, and LP-1. Following the determination of the amino acid composition, the peptide's molecular weight was further examined using HPLC. Subsequently, the peptide's sequence was ascertained through MS or MS/MS. Chen et al. assessed the molecular weight distribution of peptide CSSPs by HPLC and established its sequence using the Model L-8900 high-speed amino acid analyzer[43]. Aguilar-Toalá & Liceaga et al. identified the CSPs peptide sequence by liquid chromatography-mass spectrometry/mass spectrometry (LC-MS/MS) and also determined its molecular weight distribution[35]. In the case of peptides WPK and AYLH, their analysis involved electrospray ionization mass spectrometry (ESI-MS) to ascertain relative molecular masses. This was further refined by comparing secondary mass spectra and corroborating the peptide sequences using LC-MS/MS[36].
In addition, the characterization of peptide structures often involves nuclear magnetic resonance spectroscopy (NMR), Fourier-transform infrared absorption spectroscopy (FT-IR), and ultraviolet absorption spectroscopy (UV). Tito et al. analyzed the amino acid composition of the peptide LjSEC-EP with the biochrom 30 amino acid analyzer and further investigated its structure with MS to NMR[38]. In 2020, Li et al. structural characteristic peptides, SWP I/II, were performed by FT-IR, and UV and determined their amino acid composition by RP-HPLC[42]. Recently, Ishiguro et al. employed fast atom bombardment-mass spectrometry (FAB-MS) alongside NMR to characterize Naturido[41]. Gel permeation chromatography is used in certain studies to measure the average molecular weight of peptides. Lee et al. utilized this technique to determine the average molecular weight of the peptide CPNS, after which they examined the peptide's amino acid composition and sequence using LC-MS/MS[23].
In summary, FT-IR provides valuable information about functional groups and chemical bonds. UV spectroscopy is a rapid method for measuring concentration and identifying compounds with chromophores. NMR provides detailed structural information for molecules in solution. MS accurately determines the molecular mass and is highly sensitive for trace analysis. MS/MS provides precise structural information and identifies components in complex mixtures. LC/MS combines separation capabilities with mass determination for analyzing complex mixtures. By combining these methods, the unique characteristics of anti-aging peptides can be efficiently identified in terms of their structure and composition.
-
Neurodegenerative diseases represent significant age-related conditions wherein the brain sustains damage due to oxidative stress and inflammation (Table 2). The release of chemotactic and pro-inflammatory agents can trigger the accumulation of amyloid β-protein (Aβ), contributing to hippocampal degradation and subsequently leading to neurodegenerative disorders like Alzheimer's disease (AD) and Parkinson's disease[63−66]. Neuroimaging primarily reveals brain dysfunction, encompassing diminished spatial exploration, physical activity, spatial and non-spatial learning, memory capabilities, and anxiety-like behaviors[36,67]. The principal contributors to brain aging involve the excessive generation of free radicals and the buildup of peroxides. These factors disrupt the redox homeostasis within the internal milieu, resulting in neuronal apoptosis, microgliosis, heightened Aβ levels, decreased astrocyte count, diminished synthesis of acetylcholinesterase (AChE), and reduced activity of acetylcholine transferase (ChAT)[26,41,68−70] (Fig. 2).
Table 2. Summary of natural food-derived anti-aging peptides and aging-related diseases, models, and mechanisms.
Species Sources Peptides Aging model Delivery ways Diseases Signaling pathway Indicators References Fungus Isaria japonica Naturido SAMR1; SAMP8; Cerebral astrocytes; Hippocampal neurons; Microglial 25 μg/kg/d, 5 weeks;
25 μM, 24 h; 0.1 μM, 3 d; 1 uM, 24 hNeurodegenerative disease Inflammation AChE↓, VGF↑, NGF↑ [41] Pyropia yezoensis PYP PHNs, Glutamate, 100 uM, 60 min 1 μg/mL, 24 h Neurodegenerative disease Oxidative stress TrkB↑, PI3K↑, ERK 1/2↑, JNK↓, GRP78↓, NMDA↓, SA-β-GAL↓ [40] Pleurotus abalonus PPC SAM 30 mg/kg Enhance stress resistance Oxidative stress SOD↑, GSH-Px↑, CAT↑, MDA↓ [39] Plant Soybean WPK; AYLH Kunming mice, D-galactose,
500 mg/kg/d, 28 d; PC12 cells, Hydrogen peroxide, 0.4 mM/3 h600 mg/kg/d, 28 d;
0.05 mM, 24 hNeurodegenerative disease Oxidative stress SOD↑, GSH-Px↑, MDA↓, ROS↓, DPPH↓, AGEs↓ [75] Pea (Pisum sativum) Pep_RTE626 HDFs; Keratinocytes, Cratch cells 0.5 μg/mL, 24 h; 0.5 μg/mL, 72 h Skin aging Collagen synthesis CP↑, EP↑ [37] Cardamine violifolia CSP SD rat, Intraperitoneal injection of D-galactose, 200 mg/kg, 8 weeks 14.2 mg/kg, 8 weeks Neurodegenerative disease, Metabolic abnormalities Oxidative stress, Inflammation, Apoptosis SOD↑, GSH-Px↑, CAT↑, MDA↓, TAOC↑, ROS↓, Nrf2↑, HO1↑, NQO1↑, TNF-α↓, IL-6↓, NFkβ p65↓, AChE↓, Na+/K+-ATPase↑, p-JNK↓, RAGE↓, BACE 1↓, PS1↓, Bax↓, Bcl2↑, Caspase-3↓ [33] Rice bran KF-8 ICR mice, D-galactose, 25 mg/kg, 12 weeks; NIH/3T3 cells, Hydrogen peroxide, 100 μg/mL, 48 h 30 mg/kg, 12 weeks; 30 μg/mL, 4 h Metabolic abnormalities Oxidative stress, Inflammation, Apoptosis SOD↑, GSH-Px↑, MDA↓, Nrf2↑, Keap1↓, 8-ODdG↓, ROS↓, NF-kB p65↓, TLR4↓, lkB↓, IKK↓, MyD88↓, TNF-α↓, RAGE↓, MAPK p38↓, Bax↓, Bcl-2↑, PARP↓, Caspase-8↓, Caspase-3↓ [34] Chia seed (Salvia hispanica L.) CSPs Elastase; Collagenase; Tyrosinase; Hyaluronidase None Skin aging None Elastase↓, Collagenase↓, Tyrosinase↓, Hyaluronidase↓ [35] Lotus japonicus somatic embryo LjSEC-EP HDFs, Hydrogen peroxide,
200 uM, 2 d2 μg/mL, 24 h Skin aging Collagen synthesis GDF-11↑, Smad2↑, Collagen I↑, Collagen III↑, Periostin↑ [38] Rapeseed meal RP-1 Kunming mice, Subcutaneous injection of D-galactose,
500 mg/kg/d, 28 d600 mg/kg/d, 28 d Neurodegenerative disease Oxidative stress SOD↑, GSH-Px↑, MDA↓, DPPH↓, Na+/K+-ATPase↑, Ca2+/Mg2+-ATPase↑ [54] Potato (Solanum tuberosum L.) APPH SD rat, High fat diet, 60% equivalent, 8 weeks 15 mg/kg/d, 30 d Metabolic abnormalities Apoptosis, Inflammation Fas↓, FADD↓, Bax↑, PI3K↑, AKT↑, Caspase-3↓ [31] Potato PHP Keratinocytes 0.5% concentration,
48 hSkin aging None Cholesterol↑, Alpha-Hydroxy Fatty Acids↑, Fatty Acids↑ [53] Angelica sinensis AsiPeps Caenorhabditis elegans, Paraquat, 70 mM, 2 d 0.5 mg/mL, 24 h Skin aging Oxidative stress SOD↑, GSH-Px↑, CAT↑, MDA↓, Pigment↓ [32] Black soybean (Glycine max) BSPs Kunming mice, Intraperitoneal injection of D-galactose,
400 mg/kg, 6 weeks500 mg/kg/d, 3 weeks Enhance stress resistance Oxidative stress SOD↑, GSH-Px↑, MDA↓ [30] Animal Whey WHP C57BL/6N mice, Intraperitoneal injection of D-galactose,
100 mg/kg, 6 weeks1.5 g/kg, 30 d Neurodegenerative disease, Metabolic abnormalities Oxidative stress, Inflammation SOD↑, GSH-Px↑, MDA↓, PCO↓, TNF-α↓, IL-1β↓, AChE↓, ChAT↑, CREB↑, CaMKII↑, BDNF↑ [26] Whey WHP SAMP6 18.7% concentration, 28 weeks Metabolic abnormalities Inflammation AMPK↓, ACC↓ [83] Whey WHP HRM, Ultraviolet, 36-180 mJ/cm², 17 weeks 400 mg/kg, 17 weeks Skin aging Oxidative stress MMP2↓, MMP9↓, VGEF↓,
Ki-67↓, 8-OHdG↓, ROS↑,
ST↓, Elasticity↑, Pigment↓, Wrinkle↓[75] Whey BWW CaCo2 cells, Hydrogen peroxide, 50 mM, 30 min 0.08 mg/mL, 24 h Anti-cancer Apoptosis HSP-70↓, HSP-90↓, AKT↓, Cyclin A↓, MSA↓ [28] Collagen PCP PCFs and Keratinocytes Mitomycin C, 7.5 μg/mL, 2 h (isolated from
human)10 g, 24 h Skin aging Collagen synthesis Ki-67↑, Collagen↑ [25] Collagen CP; EP BALB/c-nude mice, Subcutaneous injection of D-galactose and Ultraviolet, 10% concentration 1g/kg and 50 mJ/cm²/30 min,
42 d and 42 d0.03 g/kg, 42 d Skin aging Collagen synthesis MMP-3↓, IL-1α↓, HYP↑, HA↑, IGF-1↑, LOX↑, Smad2↑, JNK↑, SP-1↑, TβRII↑, TGF-β↑ [62] Collagen CP Kunming mice, 2 and 13 months 400 mg/kg/d, 8 weeks Skin aging Oxidative stress, Collagen synthesis SOD↑, CAT↑, MDA↓ROS↓, ABTS↓, HA↑, Collagen I↑, Collagen III↑, DSL↓ [22] Gadus morhua SWPI; SWPII 2BS cells, Hydrogen peroxide,
0.2 mM, 2 h100 µg/mL, 24 h Enhance stress resistance Oxidative stress, Apoptosis SA-β-GAL↓, ROS↓,
Fe2+-chelating activity↑[42] Crimson snapper CSSPs Drosophila melanogaster, High fat diet, 10% (w/v), 7 d 6 mg/mL, 7 d Enhance stress resistance Oxidative stress SOD↑, CAT↑, MDA↓, PCO↓, ROS↓ [43] Fish FHS C57Bl/6J mice, 7 weeks and 12 months; BV2 cells, Lipopolysaccharide, 1 μg/mL, 6 h; HT22 cells, Lipopolysaccharide, 1 μg/mL, 6 h 5.5 mg/d, 3 months;
0.27 mg/mL, 16 h; 0.27 mg/mL, 16 hNeurodegenerative disease Inflammation NF-kB↓, IBA1↓, CD11b↓,
IL-6↓, IL-1β↓, TNF-α↓,
BDNF↑, NGF↑, Cort↑[67] Fish CPNS HRM, Ultraviolet, 55 mJ/cm2, increasing by 55mJ/cm2 every week to 220 mJ/cm2, 12 weeks; HDFs, Ultraviolet, 25 mJ/cm²/none; SD rat, 6 weeks 300 mg/kg/d, 12 weeks; 50 μg/mL, 24 h Skin aging Collagen synthesis MMP-1↓, Collagen I↑, Wrinkle↓, ET↓, SH↑, TSWL↓ [23] Oncorhynchus keta MCP C57BL/6J mice, 20 months 0.44% concentration,
3 monthsNeurodegenerative disease Oxidative stress SOD↑, GSH-Px↑, MAD↓, BDNF↑, PSD95↑ [27] Apostichopus japonicus AJPH Caenorhabditis elegans, Paraquat, 2 mM, 48 h; PC12 cells, Paraquat,
1 mM, 12 mg/mL, 10 d; 0.4 mg/mL, 24 h Enhance stress resistance Oxidative stress SOD↑, CAT↑, MDA↓, ROS↓, Pigment↓ [47] Stichopus variegates SVPF Kunming mice, Intraperitoneal injection of D-galactose,
100 mg/kg, 8 weeks; Drosophila melanogaster, D-galactose,
40 g/L, Until death1,000 mg/kg, 8 weeks;
4 g/L, Last until all
deathEnhance stress resistance Oxidative stress SOD↑, GSH-Px↑, MDA↓, PCO↓, Klotho↑ [48] Royal jelly and Carcharhinus falciformis ERJ-CP Drosophila melanogaster, Hydrogen peroxide, and Paraquat, 30% and 20 mM, 8 h and 3 d 3 mg/mL, 10 d Enhance stress resistance Oxidative stress SOD↑, GSH-Px↑, CAT↑, MDA↓, PCO↓, ROS↓ [29] Locusta migratoria manilensis LP-1 Caenorhabditis elegans, Paraquat and High temperature and Ultraviolet, 1 mM and 35 °C and 1,000 J/m², 4 h, 10.2 h and 30 s 2.5 mg/mL, 3 d Enhance stress resistance Oxidative stress ROS↓, DAF-16↑, HSF-1↑,
JNK-1↑[24] Mytilus edulis MP ICR mice, Subcutaneous injection of D-galactose, 200 mg/kg,
8 weeks1,000 mg/kg, 8 weeks Metabolic abnormalities Oxidative stress SOD↑, GSH-Px↑, MDA↓, PPARα↑, PPARγ↑, p21↓,
BS↓, Trig↓, FFA↓, HDL↑, LG↑[21] Bombyx mori SP-NN; SP-PN SD rat, Subcutaneous injection of D-galactose, 150 mg/kg, 4 weeks 50 mg/kg, 5 weeks Neurodegenerative disease, Metabolic abnormalities Oxidative stress ChAT↑, HGCP↓ [20] '↑' represents up-regulation, and '↓' represents down-regulation. The main abbreviations in Table 2 are listed in Supplemental Tables S1 & S3. SAMR: Senescence-accelerated mouse resistance; SAMP: Senescence-accelerated mouse prone; SAM: Senescence-accelerated mice; PHNs: Primary hippocampal neurons; HDFs: Human dermal fibroblasts; HRM: Hairless mice; PCFs: Primary cutaneous fibroblas. Figure 2.
Changes in related indicators of the brain during aging. ChAT, Acetylcholine transferase; ROS, Reactive oxygen species.
Contemporary strategies for the prevention and treatment of neurodegenerative diseases can be classified as follows: (i) Enhancing neuronal activity and growth while reducing apoptosis; (ii) Stimulating astrocyte proliferation; (iii) Inducing a shift in microglial phenotype towards an anti-inflammatory state or suppressing excessive microglial proliferation; (iv) Elevating levels of neuro-promoting hormones and nutritional factors that regulate hypothalamic stress; (v) Inhibiting the activity of AChE and ChAT; (vi) Reinforcing the antioxidant defense system to counteract active free radicals; (vii) Mitigating protein and lipid oxidation, curtailing pigment accumulation, and mitigating DNA damage. The 4-peptide Naturido derived from Isaria japonica significantly ameliorated age-related deficits in accelerated-aging mice via promoting astrocyte proliferation, microglial phenotype, and neuronal growth, consequently[41]. WHP treatment was shown to promote synaptic plasticity-related proteins like phosphorylated-Calmodulin-dependent protein kinase II (p-CaMKII), brain-derived neurotrophic factor (BDNF), and cAMP-response element-binding protein (CREB), which play roles in aging-related cognition, learning, and memory, in D-galactose-induced C57BL/6N mice[26]. SP could ameliorate acetylcholine depletion, brain damage, and cognition impairments induced by D-galactose in Sprague-Dawley (SD) rats. This effect was attributed to the upregulation of ChAT expression and the suppression of hippocampal astrocyte dysfunction[20].
Skin aging
-
Skin aging is the most conspicuous manifestation of aging within the body, characterized by an increase in skin thickness, pigmentation, and a decrease in skin elasticity (Table 2). The principal factors contributing to skin aging encompass the generation of oxidative free radicals, the accumulation of peroxides, the decline in antioxidative enzyme levels, and the heightened expression of diverse enzymes responsible for the hydrolysis of extracellular matrix components such as collagen and elastin[71−73]. Furthermore, senescent fibroblasts exhibit reduced capacity to synthesize collagen, elastin, and structural fibrillin, as well as produce hyaluronic acid and hydroxyproline[73]. The aging process of the skin can also impede the body's ability to effectively heal following damage[74] (refer to Fig. 3).
Figure 3.
Main causes of skin aging and related mechanisms. HA, Hydrated acid; Hyp, Hydroxyproline; SOD, Superoxide dismutase; CAT, Catalase; GSH-Px, Catalase; ROS, Reactive oxygen species; MDA, Malondialdehyde; MMPs, Matrix metalloproteinases; PCO, Pest Console Operation.
Strategies to counteract skin aging are categorized into the following approaches: (i) Enhancing levels of collagen, elastin, hyaluronic acid, and hydroxyproline; (ii) Stimulating scaffold protein fibrillin to fortify the dermal elastic fiber network; (iii) Suppressing activities of enzymes such as elastase, collagenase, tyrosinase, hyaluronidase, and matrix metalloproteinases (MMPs); (iv) Reinforcing the antioxidative defense system to mitigate the impact of active free radicals. Pep_RTE626 could ameliorate skin aging by augmenting collagen synthesis in dermal fibroblasts, fortifying the dermal elastic fiber network, and reducing elastic fiber loss[37]. CSPs could restrain the activity of elastase, collagenase, tyrosinase, and hyaluronidase associated with the prevention of skin aging[35]. WHP could mitigate the degradation of type IV collagen, curb vascular endothelial proliferation, and reduce DNA damage, leading to increased skin elasticity, decreased skin thickness, and inhibition of wrinkle formation and melanin generation[75]. According to Zhang et al., administering a combination of CP and EP through gavage resulted in elevated collagen and elastin levels in mice skin. This effect was achieved by up-regulating factors related to collagen synthesis and down-regulating the expression of MMP-3 and interleukin-1α (IL-1α)[62]. Mistry et al. have discovered that CP could significantly stimulate the proliferation of primary cutaneous fibroblasts and keratinocytes, thereby promoting skin wound healing[25]. Tito et al. have elucidated that LjSEC-EP could increase the expression of growth differentiation factor-11 (GDF-11) and that assembly of collagen in aged fibroblasts[38].
Metabolic abnormalities
-
The aberrant aging process in the human body can disrupt the equilibrium within the organism's microenvironment, subsequently contributing to metabolic dysbiosis and triggering the emergence of metabolic disorders (Table 2). These disorders encompass non-alcoholic fatty liver disease (NAFLD), diabetes, obesity, and hypertension. Such conditions arise from the deregulated regulation of blood lipids and blood glucose levels[76,77]. NAFLD is characterized by excessive fat deposition within the liver, leading to hepatocyte apoptosis due to liver inflammation and subsequent fibrosis[78−80] (Fig. 4). Anti-aging peptides have the potential to regulate NAFLD by curbing fat accumulation and inhibiting crucial proteins involved in apoptotic signal transduction. Chiang et al. documented that the peptides APPH derived from Solanum tuberosum L. could effectively suppress lipid accumulation in liver tissue of rats induced by a high-fat diet through oral administration. APPH mitigates the risk of NAFLD by modulating the phosphoinositide 3-kinase (PI3K)/AKT cascade, consequently activating cell survival markers such as factor-associated suicide (Fas), Fas-associating protein with a novel death domain (FADD), Bcl2-Associated X (Bax), and caspase-3 within liver tissue[31].
Figure 4.
Metabolic-related diseases caused by aging. (a) Non-alcoholic fatty liver disease. (b) Atherosclerosis. (c) Type 2 diabetes mellitus. (d) Fat deposition in skeletal muscle. FADD, Fas-associating protein with a novel death domain; Fas, Factor associated suicide; Bax, Bcl2-Associated X; PI3K, Phosphoinositide 3-kinase; AKT, Protein kinase B; ABCA1, ATP-Binding cassette transporter A1; ABCG1, ATP-Binding cassette transporter G1; HDL-C, High-Density lipoprotein-cholesterol; IL-6, Interleukin-6; IL-10, Interleukin-10; ACC, Acetyl-CoA carboxylase; FAS, Fatty acid synthase; AMPK, AMP-Activated protein kinase.
Aging is linked to the reduction of muscle mass in vivo, resulting in decreased metabolic capacity, and ultimately contributing to the onset of metabolic disorders like obesity and osteoporosis[81,82] (Fig. 4). Research suggested that WHP could elevate fatty acid oxidation and counteract the decline in muscle mass in senescence-accelerated mouse-prone (SAMP) 6 mice through oral administration[83]. Additionally, WHP had been found to elevate levels of high-density lipoprotein-cholesterol (HDL-C), cholesterol transport proteins ATP-binding cassette transporter A1 (ABCA1), ATP-binding cassette transporter G1 (ABCAG1), while concurrently inhibiting the expression of acetyl-CoA carboxylase alpha (ACC), fatty acid synthase (FAS), and the secretion of pro-inflammatory cytokines[84]. Studies have demonstrated that SP can enhance glucose uptake by increasing the expression of glucose transporter type 4. It also improves insulin sensitivity and enhances pancreatic β-cell activity. This effect can alleviate symptoms associated with diabetes[85,86].
Current strategies targeting aging-related metabolic disorders encompass the following approaches: (i) Mitigating blood glucose irregularities; (ii) Addressing dyslipidemia; (iii) Palliating liver dysfunction; (iv) Alleviating pathological weight loss and organ damage; (v) Enhancing antioxidant defense system by increasing antioxidant enzyme activity and reducing active free radicals; (vi) Reducing protein and lipid peroxidation, pigment accumulation, and DNA damage. A study demonstrated that MP could mitigate metabolic irregularities in a D-galactose-induced aging-mice model[21]. small molecule 8-peptide KF-8 from rice bran could prevent weight loss and enhance organ coefficients in D-galactose-induced mice, resulting in an ameliorative effect on metabolic irregularities[34].
-
Tyrosine kinase receptor B (TrkB) is known to engage in cross-talk with the PI3K/Extracellular signal-related kinases (ERK) 1/2 pathway to foster cell growth and survival[87,88]. Accumulating evidence has shed light on the role of anti-aging peptides in modulating the TrkB/PI3K/ERK 1/2 axis through binding to TrkB receptors. This modulation effectively impedes oxidative stress and cellular senescence[89,90]. The endoplasmic reticulum holds a pivotal role in maintaining intracellular calcium ionic equilibrium and becomes implicated in neurodegenerative diseases under sustained stress. PYP could activate the proliferation of primary hippocampal neurons in glutamate-induced E18 rat embryos by mediating the TrkB/PI3K/ERK 1/2 cascades. This study further revealed that PYP hindered the phosphorylation of JNK by activating the TrkB/PI3K/ERK 1/2 pathway, subsequently inhibiting the expression of Glucose-regulated protein 78 (GRP78), an individual belonging to the family of heat shock proteins located within the endoplasmic reticulum[40,91] (Fig. 5).
Figure 5.
Oxidative stress-related signaling pathways in which natural food-derived peptides exert anti-aging activity. Italics indicate genes. NFDP, Natural food-derived peptides; ROS, Reactive oxygen species; OS, Oxidative stress; Glu, Glusate; D-gal, D-galactose; Trkb, Tyrosine kinase receptor B; IGF-1, Insulin-like growth factor 1; NMDA, N-methyl-D-aspartic acid receptor; Crq, Stimulating scavenger-receptor; TLR4, Toll-like receptors 4; PI3K, Phosphoinositide 3-kinase; ERK, Extracellular signal-related kinases; JNK, c-Jun N-terminal kinase; JAK, Janus kinase; upd3, Cytokine; Kqap1, Kelch-like ECH-associated protein 1; Nrf2, Nuclear factor-erythroid 2-related factor 2; HO-1, Heme oxygenase-1; NQO1, NADPH quinone dehydrogenase 1; HSF-1, Heat-shock transcription factor-1; DAF-16, Transcription factors; AP-1, Activator protein-1; AREs, Advanced glycation end products.
Nuclear factor-erythroid 2-related factor 2 (Nrf2) plays a crucial role as a transcription factor in protecting cells from oxidative stress and regulating the expression of genes associated with antioxidants[92]. Under oxidative stress, Nrf2 dissociates from its negative regulator Keap1 and engages in nuclear interaction with antioxidant response elements (ARE). This leads to the activation of target genes in the Nrf2/ARE axis, including heme oxygenase-1 (HO-1), NADPH quinone dehydrogenase 1 (NQO1), superoxide dismutase (SOD), and glutathione peroxidase (GSH-Px), which collectively work to mitigate the impact of ROS[93,94]. CSP could increase the activity of antioxidant enzymes SOD, GSH-Px, and catalase (CAT) in D-galactose-induced SD rats. Simultaneously, it also promoted the activity of total antioxidant capacity (TAOC) and reduced the content of malondialdehyde (MDA) by activating the Nrf2/HO-1/NQO1 signaling pathway[33]. KF-8 could ameliorate aging and oxidative stress-related organ damage by promoting the Nrf2 cascade in D-galactose-induced mice. Their findings suggested that KF-8 disrupted the covalent connections between Nrf2 and Keap1 adducts or disulfide bonds, thus facilitating Nrf2 dissociation from Keap1 and enabling its nuclear translocation[34] (Fig. 5).
Dysregulated JAK/STAT, primarily responsible for orchestrating glucose metabolism in vivo, is inextricably associated with metabolic abnormalities. Studies showed that a lipid-rich diet decreases insulin sensitivity in drosophila resulting in a shorter lifespan[95,96]. In a lard-induced Drosophila aging model, CSSPs stimulated the expression and activity of antioxidant-related genes such as SOD1, SOD2, and CAT, and reduced levels of peroxide MAD and pest console operation (PCO) may through the JAK/STAT axis[43] (Fig. 5).
FOXO/DAF-16 are the key transcription factors of Insulin/Insulin-like growth factor-1 (IGF-1) signaling (IIS) in regulating biological lifespan[97,98]. The nuclear translocation of DAF-16 is primarily induced by the JNK-1 signaling pathway[99]. Within the nucleus, DAF-16 can form a complex with heat-shock transcription factor-1 (HSF-1), fostering co-activation of IIS signaling. This synergy enhances protein homeostasis and activates genes relevant to lifespan[100,101]. In the work of Cao et al. it was demonstrated that treatment with a concentration of 2.5 mg/mL LP-1 led to a 23.5% extension in the lifespan of Caenorhabditis elegans. The mechanistic insight revealed that LP-1 activated the JNK-1/DAF-16 pathway, thereby retarding the aging process in Caenorhabditis elegans exposed to oxidative stress, heat stress, and UV radiation[24] (Fig. 5).
Inflammation-related signaling pathways
-
Toll-like receptors (TLR)/Nuclear factor kappa-B (NF-κB) signaling serves as a canonical axis in regulating the inflammatory response. Research has demonstrated that excessive NF-κB activation contributes to several age-related alterations, including cognitive decline and muscle weakness in mice[102]. Upon activation, TLR4 prompts the release of NF-κB from IκBα, leading to its nuclear translocation. Pro-inflammatory factors such as LPS, D-galactose, or oxidative stress can heighten ROS levels and activate the TLR/NF-κB pathway, thereby expediting the aging process[103−105]. In a D-galactose-induced mice model, KF-8 was observed to mitigate age-related weight loss, enhance organ coefficients, and counteract aortic and brain tissue damage. This effect was attributed to KF-8's ability to inhibit proinflammatory cytokines and ROS through the suppression of the TLR4/NF-κB pathway[34]. Through the inhibition of the NF-κB pathway, supplementation of fish hydrolysate (FHS) has shown potential in reducing neuroinflammation and increasing the levels of neural factors such as nerve growth factor (NGF) and brain-derived neurotrophic factor (BDNF) both in vivo and in vitro[67]. CSP down-regulated the expression of TNF-α and IL-6 by inhibiting the NF-κB pathway in aging SD rats[26] (Fig. 6). In our recent research, flaxseed-derived linusorbs have been found to possess strong anti-inflammatory effects by inhibiting the TLR4/NF-κB/MAPK signaling pathway, which suggests that they hold significant potential for anti-aging applications[106].
Figure 6.
Inflammation-related signaling pathways in which natural food-derived peptides exert anti-aging activity. NFDP, Natural food-derived peptides; ROS, Reactive oxygen species; AGEs, Advanced glycation end products; LPS, Lipopolysaccharide; D-gal, D-galactose; RAGE, Receptor for advanced glycation end products; MyD88, Myeloiddifferentiationfactor 88; IGF-1, Insulin-like growth factor 1; BACE-1, Beta-site APP-cleaving enzyme 1; Aβ-42, Amyloid β-protein; PS-1, Presenilin-1; IKK α/β/γ, IκBkinase α/β/γ; IκB, inhibitor of NF-κB; NF-κB, Nuclear factor kappa-B; BDNF, Brain-derived neurotrophic factor; PI3K, Phosphoinositide 3-kinase; AKT, Protein kinase B; TLR4, Toll-like receptors 4.
Advanced glycation end-products (AGEs) are pivotal markers in the oxidative stress process. They bind to their receptor, RAGE, leading to the overexpression of aging-associated substances like Aβ polypeptides and ROS. As age increases, the gradual accumulation of AGEs in tissues and organs perpetuates damage to these structures and neural systems[107,108]. Studies have demonstrated that AGEs/RAGE signaling can cross-talking with the NF-κB pathway resulting in activating inflammation[109,110]. Additionally, both ROS and D-galactose can trigger the accumulation of Aβ42, beta-site amyloid precursor protein-cleaving enzyme 1 (BACE1), and presenilin-1 (PS-1), provoking neuroinflammation within the brain by activating the AGEs/RAGE pathway[111−113]. The onset of Alzheimer's disease has a significant correlation with the activation of AGEs/RAGE pathway signaling, often accompanied by elevated levels of BACE1 and PS-1[114−116]. A study revealed that intraperitoneal injection of CSP significantly inhibited inflammation and aging via suppressing AGEs/RAGE pathway signaling in D-galactose-induced SD rats[33] (Fig. 6).
Apoptosis-related signaling pathways
-
The PI3K/AKT signaling pathway plays a crucial role in controlling both cell proliferation and metabolism[117]. Abnormal PI3K/AKT signaling pathways have been observed in numerous studies focusing on aging-related diseases[118−120]. Cytokines or growth factors prompt phosphorylation of receptor tyrosine kinase (RTK) and activate the PI3K/AKT pathway as a response to external environmental cues[121,122]. Multiple investigations have unveiled that natural compounds often safeguard neurons and curb microglial activation through modulation of the PI3K/AKT signaling pathway in Alzheimer's disease (AD) treatment[123−125]. In hydrogen peroxide-induced CaCo-2 cells, BWW resulted in the downregulation of HSP-70 and HSP-90 expression. Furthermore, anti-HSP expression was also curtailed (Fig. 7). BWW significantly dampened AKT activity, a downstream effector of PI3K, which is associated with colorectal cancer proliferation. Studies have also disclosed the cross-talk between PI3K/AKT signaling and NF-κB signaling, which triggers aberrant metabolisms such as liver fat accumulation, hepatocyte apoptosis, and fibrosis[126−128]. APPH could significantly suppress the expression of proinflammatory cytokines by activating PI3K/AKT signaling to reduce the high-fat diet-induced liver fat deposition, hepatocyte apoptosis, and fibrosis in rats[31] (Fig. 6).
Figure 7.
Apoptosis-related signaling pathways in which natural food-derived peptides exert anti-aging activity. NFDP, Natural food-derived peptides; ROS, Reactive oxygen species; D-gal, D-galactose; Fas, Factor associated suicide; FADD, Fas-associating protein with a novel death domain; TLR4, Toll-like receptors 4; MyD88, Myeloid differentiation factor 88; JNK, c-Jun N-terminal kinase; MAPK, Mitogen-activated protein kinases; Bax, Bcl2-Associated X; Bcl-2, B-cell lymphoma-2; HSP 70/90, Heat shock protein 70/90; AKT, Protein kinase B; PARP, Poly ADP-ribose polymerase.
The mitogen-activated protein kinases (MAPKs) signaling pathway plays a key role in regulating gene expression related to both cell proliferation and apoptosis[129]. Comprising three branches, namely ERK 1/2, JNK-1/2/3, and p38 proteins (p38 a/b/g/d), play an important role in apoptotic signal transduction in vivo[130,131]. Activation of p38 MAPK through stimulation of the TLR4 receptor augments the expression of pro-apoptotic factors, thereby fostering cell apoptosis and hastening senescence[116,132]. KF-8 exhibited potential in ameliorating damage to the aorta and brain in D-galactose-induced ICR mice. A plausible mechanism underlying this effect was the suppression of the pro-apoptotic factor Bax, achieved by inhibiting the p38 MAPK signaling pathway[34] (Fig. 7). Neuronal apoptosis induced by D-galactose may be attributed to the activation of the p-JNK pathway[133]. Oxides and peroxides also can activate JNK through the production of ROS[134]. Activated JNK, in turn, elevates caspase-3 expression and the Bax/Bcl-2 ratio, thereby expediting cell apoptosis and advancing organismal aging[135]. CSP could inhibit neurodegeneration by suppressing the phosphorylation of JNK in D-galactose-induced SD rats. Meanwhile, the level of caspase-3 and the ratio of Bax/Bcl-2 were down-regulated by CSP[33] (Fig. 7).
Fas, known as CD95, signaling pathway is one of the important pathways regulating apoptosis[136]. Upon engagement with its ligand (Fas L), Fas stimulates the propagation of apoptotic signals, ultimately culminating in cell apoptosis[137]. The activation of Fas entails a sequence of steps. Initiated by ligand binding, receptor trimerization occurs, thereby establishing an apoptosis-inducing complex on the cell membrane, comprising FADD with a death domain. After receptor-Fas interaction, the initiation of lethal signal transduction transpires, ultimately propelling cell demise[138]. A high-fat diet could re-recruit FADD molecules by activating the death receptor Fas and stimulating the cleaved form of caspase-8 and caspase-3, thereby triggering the occurrence of apoptosis[139,140]. Chiang et al. found that APPH could inhibit liver fat accumulation, hepatocyte apoptosis, and fibrosis and extend the lifespan of a high-fat diet that induces aging SD rats. The apoptotic markers, Bax, and caspase-3 were mediated through the Fas signaling pathway[31] (Fig. 7).
Collagen synthesis-related signaling pathways
-
Regulating collagen synthesis-related signaling pathways represents a principal approach in retarding skin aging, with the TGF-β/Smad axis serving as a pivotal pathway governing cellular growth and differentiation[141]. TGF-β orchestrates collagen-associated gene expression by binding to TGF-β receptor II (TβRII) and activating nuclear translocation of Smad-2[142]. Growth differentiation factor 11 (GDF 11), a member of the TGF-β family, also advances collagen expression and the construction of extracellular matrix (ECM) structures through the GDF11/Smad cascade[143]. LjSEC-EP promoted collagen synthesis and restored expression of GDF-11 in H2O2-induced senescent fibroblasts by mediating the TGF-β/Samd signaling pathway[38]. In a study by Zhang et al., administering a mixture of CP and EP at 0.03 g/kg to BALB/c-nude mice notably upregulated collagen and elastin levels in the animals' skin. This enhancement in collagen and elastin was mediated through the expression modulation of seven relevant factors: IGF-1, lipoxygenase (LOX), Smad-2, JNK, SP-1, TβRII, and TGF-β. These factors collectively contributed to regulating the ECM's integrity, consequently fostering skin repair[62] (Fig. 8).
Figure 8.
Collagen synthesis-related signaling pathways in which natural food-derived peptides exert anti-aging activity. Italics indicate genes. NFDP, Natural food-derived peptides; GDF-11, Growth differentiation factor-11; TGF-β, Transforming growth factor-β; Smad, Small mothers against decapentaplegic; TNF-α, Tumor necrosis factor-α; IL-1α, Interleukin-1α; MMP, Matrix metalloproteinase; JNK, c-Jun N-terminal kinase; LOX, Ysyloxidase; IGF-1, Insulin-like growth factor 1; TβRII, Transforming growth factor-β receptor II; SP-1, Transcription factor SP-1.
-
Numerous bioactive peptides derived from food sources have been identified and shown to exhibit diverse pharmacological effects, including antimicrobial, antihypertensive, antioxidant, anti-obesity, blood-lipid-lowering, and anti-aging activities[144−146]. This review article delves into the anti-aging potential and associated mechanisms of these bioactive peptides derived from food sources. The focus encompasses their roles in mitigating aging-associated conditions such as neurodegenerative diseases, skin aging, and metabolic irregularities[26,36,67]. These functionally active peptides are typically comprised of fewer than 20 amino acids and possess a molecular weight below 8 kDa. This structural characteristic underlies their high bioavailability upon ingestion. Notably, the peptides mentioned possess potent reactive oxygen species and reactive nitrogen species scavenging abilities, which can be attributed to the presence of polar and aromatic amino acids within them, thereby qualifying them as potential functional dietary supplements or therapeutic agents for combating the aging process[29,30,38,43].
As discussed earlier, these peptides derived from food sources exhibit distinct anti-aging capabilities in vivo, exerting their effects through various mechanisms: (1) 'Neurodegenerative Diseases': These peptides promote the proliferation and survival of neurons, thereby improving neurodegenerative conditions. This is achieved through the modulation of signaling pathways associated with apoptosis[26,41,67]; (2) 'Skin Aging': Food-derived peptides activate the biosynthesis of collagen and elastin, while concurrently inhibiting genes responsible for collagen degradation. This dual action contributes to the mitigation of skin aging in vivo[35,62,75]. (3) 'Metabolic Disorders': These peptides regulate lipid and glucose metabolism, leading to the alleviation of metabolic disturbances and subsequently delaying the aging process[33,34,83]. It is important to note, however, that the anti-aging effects of these peptides have primarily been demonstrated in animal models such as rodents, Drosophila, and Caenorhabditis elegans. The translation of these effects to humans, along with the underlying mechanisms, remains uncertain and warrants investigation through human clinical trials. Additionally, the specific target markers and cellular networks affected by these food-derived anti-aging peptides in vivo have yet to be fully elucidated[36,37,48]. To further advance our understanding, ongoing research efforts should aim to uncover the precise mechanisms of action of these anti-aging peptides, providing a solid foundation for their potential application in the development of anti-aging interventions.
We propose strategies for using food-derived anti-aging peptides in the food industry, focusing on the following areas: (1) Selecting suitable ingredients: Choose food ingredients rich in anti-aging peptides, such as marine organisms, plant seeds, and animal proteins, which contain stable and biologically active anti-aging peptides. (2) Optimizing processing techniques: Use appropriate methods like ultrasound, high-pressure treatments, enzymatic hydrolysis, or fermentation to preserve and extract anti-aging peptides during food processing, maximizing their efficiency and stability. (3) Ensuring product quality and safety: Maintain strict control over the sourcing and processing of raw materials to prevent contaminants and harmful substances. Implement proper storage and packaging methods to extend shelf life and preserve peptide activity. (4) Conducting effective clinical studies: Perform clinical research to validate the efficacy and safety of food-derived anti-aging peptides in humans. This will provide scientific data to assess their impact on human health and determine appropriate dosages and administration methods. Currently, certain anti-aging peptides have found application in the cosmetics industry. Their primary function lies in the activation of fibroblasts, which subsequently elevates the levels of glycosaminoglycans, matrix proteins, collagen, and elastin in the dermis[147,148]. However, the effective utilization of these bioactive peptides faces two key challenges: their categorization as either nutritional components or therapeutic agents for combating aging-related ailments. When regarded as nutritional elements, the structural characteristics of these bioactive peptides render them susceptible to hydrolysis by proteases in the gastrointestinal tract. Therefore, the development of a delivery system that ensures targeted release of the peptides within the intestines, while circumventing gastric hydrolysis, becomes imperative. Conversely, if these peptides are intended for use as therapeutic agents, it becomes crucial to regulate their release to preserve their bioactivity in vivo. The successful application of anti-aging peptides as functional food components to enhance human health and maintain metabolic equilibrium, or as promising candidates for treating aging-related conditions, would represent a significant milestone. Such achievements hinge on the meticulous investigation and understanding of their behavior within the body.
-
Natural food-derived peptides obtained from animals, plants, and microbiotas have demonstrated remarkable efficacy against conditions like Alzheimer's disease, Parkinson's disease, skin aging, and metabolic disorders. These peptides function by effectively regulating oxidative stress, inflammatory responses, apoptosis, and collagen synthesis. Additionally, they have exhibited the ability to influence nutrient-sensing pathways like PI3K/AKT and Sirtuins. Nevertheless, there remains a substantial knowledge gap concerning the intricate relationship between the specific structural attributes of these natural food-derived anti-aging peptides and their mechanisms of action. Further investigations are warranted to shed light on this aspect. Moreover, exploring the potential applications of these peptides in combating aging and elucidating their effects on human health holds promising avenues for future research.
-
The authors confirm contribution to the paper as follows: writing - original draft: Li J; writing - review & editing: Wang J, Li J; resources: Zhang N; visualization: Li Y; validation: Cai Z, Liu Z; data curation: Li G, Liu Z; project administration: Wang Y, Shao X, Chen J; supervision: Shao X, Chen J; funding acquisition: Chen J. All authors reviewed the results and approved the final version of the manuscript.
-
All data generated or analyzed during this study are included in this published article and its supplementary information files.
This work was supported by the National Key R & D Program of China (Grant No. 2018YFE0108400) and Guangzhou Science and Technology Plan Project (Grant No. 201903010049) are gratefully acknowledged.
-
The authors declare that they have no conflict of interest.
- Supplemental Table S1 The full name and abbreviations of anti-aging peptides and amino acids.
- Supplemental Table S2 The full name and abbreviations of the methods for isolating, purifying, and identifying anti-aging peptides and amino acids.
- Supplemental Table S3 The full name and abbreviations of aging-related indicators.
- Copyright: © 2023 by the author(s). Published by Maximum Academic Press on behalf of China Agricultural University, Zhejiang University and Shenyang Agricultural University. This article is an open access article distributed under Creative Commons Attribution License (CC BY 4.0), visit https://creativecommons.org/licenses/by/4.0/.
-
About this article
Cite this article
Li J, Wang J, Zhang N, Li Y, Cai Z, et al. 2023. Anti-aging activity and their mechanisms of natural food-derived peptides: current advancements. Food Innovation and Advances 2(4):272−290 doi: 10.48130/FIA-2023-0028
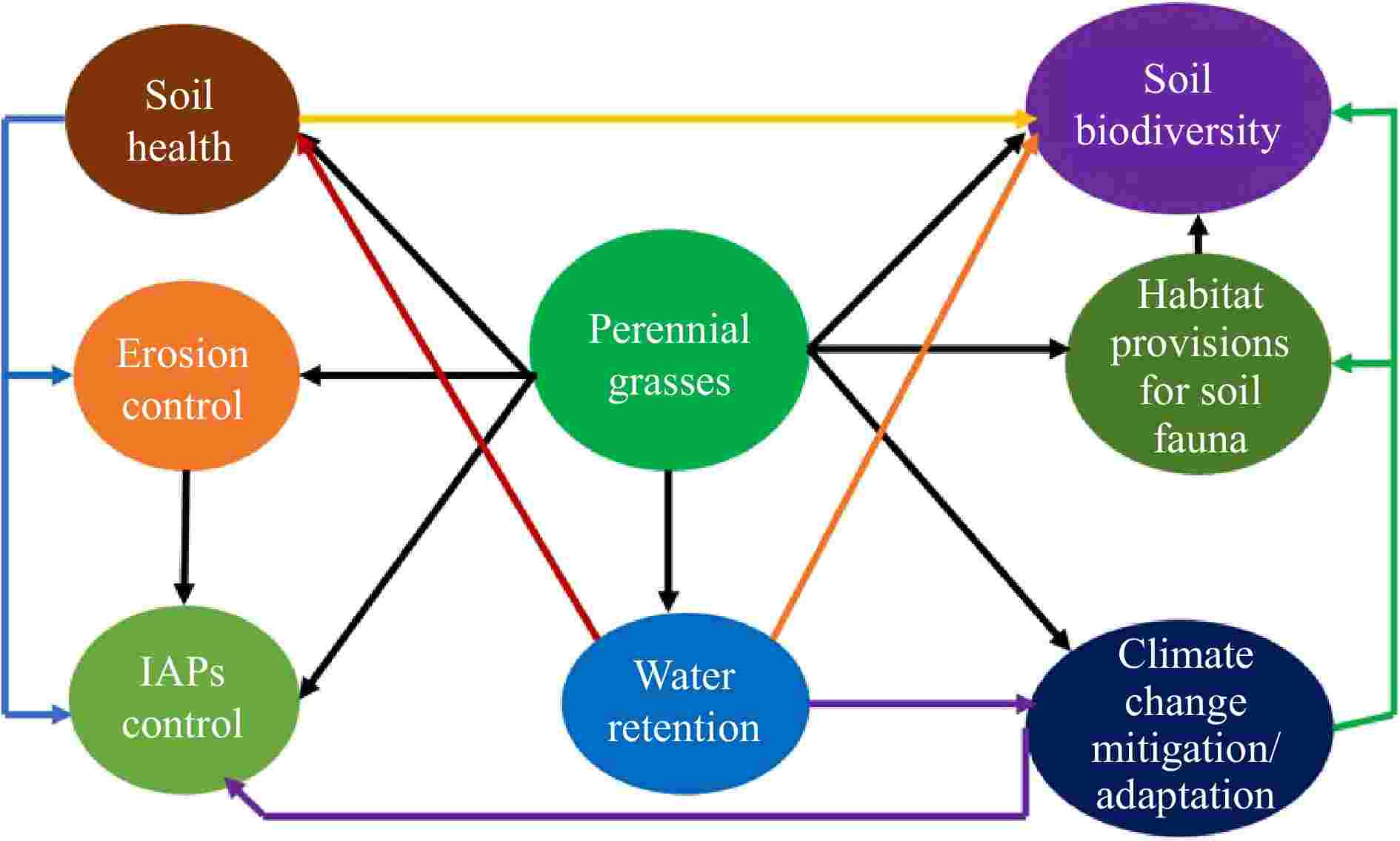