-
A medicinal plant is any plant that contains in one or more of its organs a substance that can be used for therapeutic purposes or as a precursor for the synthesis of useful drugs[1]. There are between 50,000 and 80,000 flowering plant species used for medicinal purposes worldwide[2]. Their abundant secondary metabolites (SMs), a diverse group of chemicals including alkaloids, terpenoids, glycosides, amines, steroids, flavonoids, and related metabolites, have been widely used in the drug and pharmaceutical industries[3]. To achieve high yields and quality in medicinal plants, scientists have recently studied various factors influencing these characteristics, such as environmental conditions and the plants' metabolic processes, achieving significant results. In these studies, plant small RNAs, particularly microRNAs, have been found to play a crucial role in regulating organ development and secondary metabolism in medicinal plants.
MicroRNAs (miRNAs) are produced from plant MIR genes and are non-coding RNAs, meaning they do not translate into proteins. The transcription of MIR genes by DNA-dependent RNA polymerase II results in single-stranded, polyadenylated, and self-complementary primary miRNAs, which fold into hairpin-like structures. The precursor miRNA that forms the stem-loop structure is cleaved by DCL to yield mature miRNA duplexes. One of the strands will be degraded while the duplexes are loaded onto the AGO protein to form the RISC. This complex facilitates miRNA function by binding to and cleaving the transcripts of target genes, thereby halting gene expression and serving a regulatory role[4,5]. Over the past two decades, extensive research has demonstrated the significant roles miRNAs play in various aspects of plant growth and development.
In this review, we summarize recent research on miRNAs in medicinal plants, mainly including two aspects. On the one hand, miRNA regulates the organ growth and development of medicinal plants, and on the other hand, it regulates the pathways of SMs.
-
The medicinal parts of distinct medicinal plants are discrepant. Roots, stems, leaves, flowers, fruits, and seeds can be used as phytomedicine. The growth and development regulation mediated by microRNA is manifested in various organs of medicinal plants (Fig. 1). During the development of the Panax ginseng, miR156 and miR5015 target the transcription factors SPL24-09 and ARF, respectively, to regulate the growth of adventitious roots[6]. Similarly, miR396b regulates the development of hairy root by targeting GRFs (Fig. 1)[7].
Figure 1.
MicroRNAs involved in organ development of medicinal plants. The miRNAs marked with different colors in the figure indicate their regulatory effects on different growth parts of medicinal plants: Brown miRNAs regulate root system development, green ones participate in stem morphogenesis, red miRNAs affect fruit development, blue counterparts mediate leaf development, orange markers correlate with floral organ development, while black-labeled miRNAs specifically govern the formation and developmental mechanisms of epidermal trichomes in certain medicinal plants.
The medicinal plant Zingiber officinale Roscoe is also widely used in the field of pharmacology. miR156, miR319, miR171a-2, miR164, miR529, and their respective targets transcription factors SPL, MYB, GRF, SCL, and NAC regulate the development of its rhizomes by up-regulation and down-regulation[8]. The development of Curcuma longa rhizomes is regulated by regulatory miR156-SPL and miR5015-ARF modules[9]. In Pinellia ternata, the development of its medicinally used stem tuber is regulated by miR156a-SPL, miR167a-ARF6, and miR171a-SCL6 modules[10]. In sweet potatoes (Ipomoea batatas L.), the regulatory modules miR319-TCP4 and miR172-AP2 regulate the development of sweet potato root tuber by responding to auxin signals[11]. Furthermore, miR156 targets SPL to regulate Stephania japonica development[12]. Swertia chirayita leaf development is regulated by the miR156-SPL module[13]. Car-miRN777 and miR319a regulate the flowering process of Lonicera japonica Thunb. by targeting the transcription factors ELF7/CPK33, MYB33/TCP4[14]. The regulatory module miR172-AP2 likewise regulates the flowering process of Viola prionanth[15]. Fruit growth in Lycium chinense Miller involves miR156-WRKY8, miR157-CNR, miR171-GRAS, and miR6164-NAC regulatory modules[16]. Additionally, trichomes, which serve as major secretion sites for secondary metabolites in medicinal plants (such as volatile oils), are subject to developmental regulation that may impact the quality of medicinal plants. miR390, miR160 targeting the transcription factor ARF and miR828 targeting MYB17 to regulate the development of Artemisia annua trichomes, which may affect the content of artemisinin (Fig. 1)[17−19].
-
The different kinds of SMs produced by these secondary metabolic pathways are the active ingredients of medicinal plants. Examples include tanshinone and salvianolic acid in Salvia miltiorrhiza, artemisinin in Artemisia annua, and flavonoid compounds in Scutellaria baicalensis. Current research on the regulation of the synthesis of active ingredients in medicinal plants by miRNAs mainly focuses on the shikimic acid, mevalonate, and acetate-malonate acid pathway (Fig. 2).
Figure 2.
microRNAs involved in secondary metabolite biosynthesis of medicinal plants. PAL, phenylalanine ammonia-lyase; TAT, Tyrosine aminotransferase; ACP, acyl carrier protein; FAH, fatty acid hydroxylase; LACS, long-chain acyl-CoA synthetase; DXR,1-deoxy-D-xylulose-5-phosphate reductoisomerase; DXS, 1-deoxy-D-xylulose-5-phosphate synthase; HDR, (E)-4-hydroxy-3-methylbut-2-en-1-yl diphosphate reductase; ACCT, Acetyl-CoA carboxylase; HMGR, 3-hydroxy-3-methylglutaryl-CoA reductase; DBR2, Artemisinic aldehydeΔ11 (13) reductase; PTS, patchoulol synthase; TDC, Tyrosine decarboxylase; STR, Strictosidine synthase; G10H, geraniol 10-hydroxylase; ADS, amorpha-4,11-diene synthase; T13OH, taxane 13α-hydroxylase; TBT, taxane 2α-O-benzoyltransferase, TCS1: caffeine synthase. A single star indicates that the miRNAs and its targets have been verified by quantitative real-time PCR (qRT-PCR) experiment. Two stars signify that the miRNAs and its targets have been verified by either the Rapid Amplification of cDNA Ends (RACE) or degradation group experiments. Three stars denote that the miRNAs and its targets have been verified by transgenic assays. The dashed lines indicates that there are multiple steps in this metabolic process, which are omitted to highlight the miRNAs and their targets.
miR396b targets UDP-glycosyltransferases, miR2673a targets WRKY37, MYBF1, miR828b targets phosphoenolpyruvate carboxylase, miR2910 targets malate dehydrogenase, and phe-miR1438 targets caffeoyl-CoA O-methyltransferase to regulate the synthesis of podophyllotoxin in Sinopodophyllum hexandrum (Fig. 2)[20].
Alkaloid compounds are also regulated by miRNAs. In opium poppy, pso-miR2161 and pso-miR13 target 4-OMT and 7-OMT, respectively, to regulate morphine synthesis[21]. The synthesis of piperine in Piper nigrum is mediated by miR166, miR396, and miR397, which target the key enzyme genes 4CL, PER, and CCR[22]. In the Catharanthus roseus, miR160 targets the transcription factor ARF affecting the expression of TDC, STR, and G10H; miR5021 targets GCPE to regulate the biosynthesis of vinblastine and vincristine[23,24]. Terpene alkaloids paclitaxel is the active ingredient of the plant Taxus. miR164 targets Taxane 13α -hydroxylase, and miR171 targets Taxane 2α-O-benzoyltransferases to influencing its biosynthesis[25]. miR1446a targets bHLH1, which binds to the promoter of TCS1 to affect the synthesis of caffeine in Camellia sinensis (Fig. 2)[26].
Flavonoids and organic acids are the active ingredients of Lonicera japonica Thunb. miR160I targets ADT[14], U2743257 targets 12'H to regulate the biosynthesis of isoflavones. U4992168 and miR482d targets DFR to regulate the synthesis of anthocyanins. U977315 targets ACP to regulate the synthesis of fatty acids, while U436803 and U805963 target LACS and FAH to regulate the elongation of fatty acid chains[27]. In Chrysanthemum morifolium, miR393b-p5, PC-3p-40855_284 targets PAL and 4CL to regulate the flavonoids and chlorogenic acid biosynthesis[28]. In Scutellaria baicalensis, miR858 targets the transcription factor MYB47, affecting its interaction with PAL and FNS promoters for flavonoid compound synthesis (Fig. 2)[29].
In Salvia miltiorrhiza, miR858 regulates the synthesis of salvianolic acid by targeting the transcription factors MYB6H and MYB112[30], which in turn regulate the key enzyme PAL and TAT for its biosynthesis. In addition, miR159a targeting CYP98A14 also regulates its biosynthesis[31]. In tanshinone synthesis, miR858 targets the transcription factor MYB6H and MYB112 regulate the expression of the key enzymes SmKSL1 and SmCPS1; miR396b targets SmGRFs, SmHDT1, and SmMYB37/4 to regulate its biosynthesis (Fig. 2)[7].
Terpenoid compounds are also modulated by miRNAs. Artemisinin is the active ingredient of Artemisia annua. miR159, miR172, and miR166 target a CPR gene[32]; miR160 and miR858a target transcription factors ARF1 and MYB1, which in turn affect the expression of DBR2 and ADS to regulate its biosynthesis[19]. Paeoniflorin, a monoterpene glycoside in Paeonia lactiflora. miR319a and miR156 target the transcription factors TCP2 and SPL12 to regulate the expression of the key enzyme genes TPS8 and TPS16, and miR396c, miR1144a target CYP71A1, DXS to regulate the biosynthesis of paeoniflorin[33]. Monoterpene compound Patchouli oil, as an active ingredient in Pogostemon cablin, is regulated by miR156 targeting SPL, which regulate PaPTS expression[34]. In Picrorhiza kurrooa, miR4955 targets 3-deoxy-7-phosphoheptulonate synthase to regulate the synthesis of picroside I[35]. Oridonin, the active ingredient of Isodon rubescens is regulated by stu-miR167d-3p targeting DXS, mtr-miR397 targeting HDS, and smi-miR12112 targeting HMGR[36]. miR167, novel_miR2924, and novel_miR218 regulate the synthesis of terpene trilactones in Ginkgo biloba by targeting enzymes ACCT, HMGR, and MK[37]. In Alisma orientale, aof-miR408 and Novm0007-5p target HMGR to regulate the biosynthesis of Protostane triterpenes (Fig. 2)[38].
In Actinidia chinensis and Arabidopsis thaliana, miR858 targets the transcription factor MYB to regulate the synthesis of flavanol and proanthocyanidin[39]. In Salvia miltiorrhiza and Populus trichocarpa, miR397a targets LACs to regulate the synthesis of proanthocyanidin (Fig. 2)[40].
-
It has been found in research that several miRNAs from medicinal plants can directly serve as effective substances to treat certain diseases. The plant miRNAs may regulate the expression of mammalian or virus target genes, for example, plant miR168a specifically targeting mammalian LDLRAP1[41]. Plant-derived miR159 can target the 3' UTR of transcription factors in breast cancer tissues to inhibit tumor growth[42]. Another study reported that the plant miR2911 in the decoction of Lonicera japonica can be absorbed by mice and directly target the influenza A virus, thereby inhibiting virus replication and achieving the purpose of treating diseases[43]. It is also found that the sRNA of traditional Chinese medicine can effectively inhibit the gene disorders caused by the novel coronavirus. The sRNA of Rhodiola rosea can be used for the prevention and treatment of fibroproliferative disorders and/or syndromes[44]. The potential of microRNA in medicinal plants as an active ingredient for directly treating diseases via cross-kingdom regulatory manner requires further research to understand its universality and effectiveness.
-
In conclusion, we can see that microRNA plays a crucial role in the growth, development, and secondary metabolism of medicinal plants. On one hand, miRNA targets transcription factors such as SPL and MYB to regulate the growth and development of various organs of medicinal plants, and then affects the yield of the medicinal parts of plants; on the other hand, miRNA targets the key enzyme genes and transcription factors in the active ingredient synthesis pathway of medicinal plants to regulate their biosynthesis, which has a very important role in the formation of the quality of medicinal plants. In addition, the miRNAs of medicinal plants can also be directly used as an active ingredient to treat diseases. The study of microRNA in medicinal plants has a very broad application prospect. However, at present, there is not much research in this field, only dozens, and the research is not in-depth. To date, most miRNA-mediated regulation in medicinal plants only relies on target prediction and expression profiles, only a few miRNAs’ functions were confirmed by solid experiments, such as transgene assay. In the future, studies on medicinal plants should pay more attention to neo-functionalization of conserved miRNA as well as those lineage-specific or novel miRNAs. By leveraging newly developed transgenic methods such as the 'Cut-dip-budding (CDB)'[45] system and CRISPR-Cas gene editing to clarify the biological functions of miRNAs, these key regulatory molecules can serve as powerful tools for modulating the biosynthesis of SMs and improving valuable traits in medicinal plants. For instance, the CRISPR-Cas12a nuclease can target and knockout MIR genes, disrupting the secondary structure of pre-miRNAs to block mature miRNA production[46]. This enables functional validation by observing expression changes in potential downstream targets. The application of these technologies has significantly enhanced the efficiency and precision of miRNA gene regulatory network research, with CRISPR-Cas12a achieving 100% editing efficiency, providing a robust platform for functional studies. Further optimization of experimental design and workflows will deepen insights into miRNA-mediated gene regulation mechanisms and advance their application in tailoring medicinal plant traits. On the other hand, in some cases, medicinal plant miRNA could directly serve as effective substances to treat certain diseases via trans-kingdom targeting. Based on this, conjugating miRNAs with nanoparticles or other delivery systems can enhance their stability and delivery to target tissues. Otherwise, miRNAs or even artificial miRNA could be explored as therapeutic agents or used in combination with existing therapeutic agents. Future research should focus on understanding the precise mechanisms of action, optimizing delivery methods, and conducting clinical trials to evaluate their safety and efficacy in human health, which needs interdisciplinary collaborations.
This review was supported by the Natural Science Foundation of Hubei Province (Grant No. 2024AFB731), and the Seed Industry High-quality Development Project of Hubei Province (Grant No. HBZY2023B00503).
-
The authors confirm contribution to the paper as follows: study conception and design: Liu Y, Mei Z; draft manuscript preparation: Yang Y, Chen W, Liu Y. All authors reviewed the manuscript and approved the final version of the manuscript.
-
Data sharing is not applicable to this article as no new data were created or analyzed in this study.
-
The authors declare that they have no conflict of interest.
- Copyright: © 2025 by the author(s). Published by Maximum Academic Press, Fayetteville, GA. This article is an open access article distributed under Creative Commons Attribution License (CC BY 4.0), visit https://creativecommons.org/licenses/by/4.0/.
-
About this article
Cite this article
Yang Y, Chen W, Mei Z, Liu Y. 2025. The multifaceted role of microRNA in medicinal plants. Medicinal Plant Biology 4: e009 doi: 10.48130/mpb-0025-0006
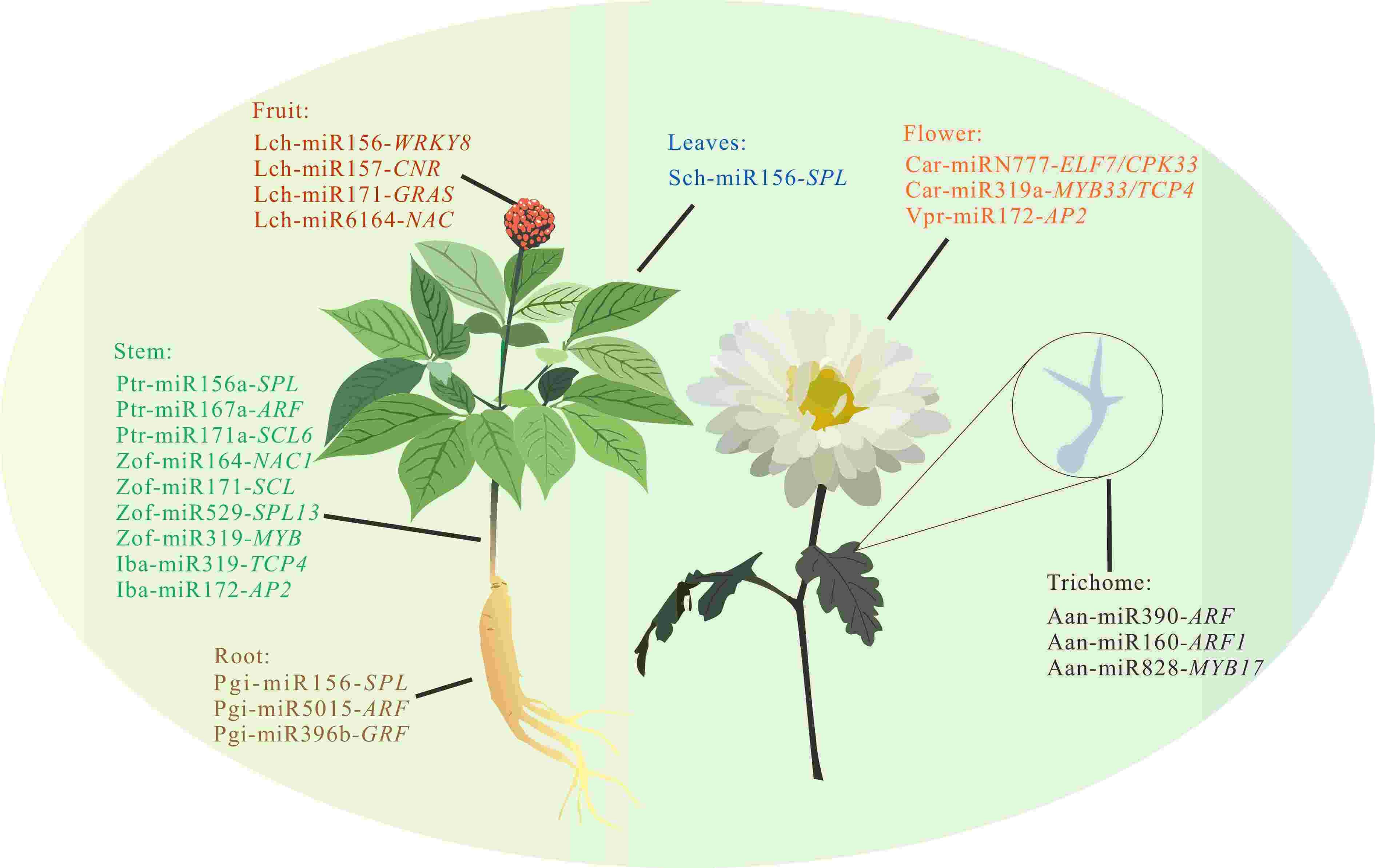