-
Diabetes mellitus (DM), a metabolic disorder characterized by an increase of blood glucose level or hyperglycemia, has been one of the principal causes of death worldwide with about 6.7 million mortalities in 2021[1]. This is compounded by the fact that 81% of DM cases were recorded in low- and middle-income countries. These alarming figures warrant scientists to research alternative drugs against the disease. The use of α-glucosidase inhibitors as antidiabetic drugs has been shown to competitively inhibit the enzyme, thereby controlling blood glucose levels. The commercial α-glucosidase inhibitor, acarbose, had been found to inhibit intestinal glucosidases[2]. However, common side effects such as flatulence and diarrhea were reported. Hence, there has been a growing number of studies to look for other safer and effective α-glucosidase inhibitors from natural sources.
The genus Pandanus is a group of tropical medicinal plants that have been used to treat diabetes in Asia and Africa. Several species of Pandanus were already reported to possess antidiabetic potential, e.g., the ethyl acetate leaf extract of Pandanus canaranus[3], the caffeoyl-quinic rich fruit of P. tectorius[4], the leaf extract of P. amaryllifolius[5,6], the aqueous root extracts of P. odorus[7], and the aqueous and ethanol prop root extracts of P. fascicularis[8]. These studies highlighted the potential of Pandanus for antidiabetic therapy.
In addition to plants, fungal endophytes, i.e., mutualistic organisms that live within healthy plant tissues, can be mined to produce chemically diverse secondary metabolites with potential pharmaceutical applications[9] including about 200 fungal secondary metabolites that had been screened and reported for their α-glucosidase inhibitory activity[10]. For instance, the fungus Talaromyces amestolkiae produced 14 isocoumarins, four of which had five-fold more potency than acarbose[11]. Furthermore, rubrolide S, a butenolide polyketide from the endophyte Aspergillus terreus, exerted a potent anti-competitive mode of inhibition against α-glucosidase[12].
Fungal endophytes from the tropical plant Pandanus have been previously reported and studied for their bioactivities. Endophytic fungi from P. amaryllifolius were collected and isolated in the Philippines[13]. New compounds with high biological activities (antibacterial, antioxidant, and anticancer) were identified, including colletotriolide from Colletotrichum sp.[14], diaportheones A and B from Diaporthe sp.[15], and guignardiol from Guignardia sp.[16]. The host plant has also shown promising antimicrobial activities[17]. These studies led us to screen fungal endophytes from another species, Pandanus simplex, for its α-glucosidase inhibitory activity. From our screening, the fungal endophyte Annulohypoxylon stygium showed promising α-glucosidase inhibition activity. The bioactive metabolite was also isolated and identified from the crude extract of A. stygium.
-
Twelve morphospecies of fungal endophytes were isolated from the mature leaves of Pandanus simplex (coded as PMEF01 to PMEF12). All morphospecies remained as mycelia sterila and did not produce any spores even after prolonged incubation. Preliminary screening of their crude culture extracts for α-glucosidase inhibitory activity showed varied activities with IC50 values ranging from 31.88 to 260 µg/mL, which was exhibited by 10 morphospecies, and with two morphospecies exhibiting > 1,000 µg/mL (data not shown). Owing to its promising result, i.e., with highest α-glucosidase inhibitory expressed as the lowest IC50 value (31.88 ± 2.86 µg/mL), the fungal endophyte PMEF05 was chosen for further study.
Colonial morphology of PMEF05 appeared as a white, filamentous colony with a distinct light brown pigmentation on the culture media (Fig. 1). PMEF05 did not sporulate even if grown on different culture media. Therefore, PMEF05 was subjected to molecular sequencing of ITS genes. Based on the comparison of the resulting sequence through BLAST, a 100% similarity between PMEF 05 and the Annulohypoxylon stygium isolate XH3 (Accession No: FJ848852) was observed. The identity is supported by our phylogenetic analysis (Fig. 1).
Figure 1.
ML tree and colony of Annulohypoxylon stygium (PMEF05), a fungal endophyte of the tropical plant, Pandanus simplex.
The crude extract of A. stygium PMEF05 was fractionated affording seven fractions and tested for its α-glucosidase inhibitory activity. Of these seven fractions, only fraction 1 displayed excellent bioactivity (IC50 79.86 ± 0.82 µg/mL); hence, was further purified to obtain a pure compound. This isolate is a light brown powder which showed a blue spot upon spraying with FeCl3-K3Fe(CN)6, thereby indicating the presence of a phenolic moiety. It also exhibited an IC50 value of 676.3 ± 1.03 µg/mL with the α-glucosidase inhibition assay.
Based on the ESI-MS spectrum of the isolated compound, the molecular ion peak at m/z 175.86 [M+H]+ (calculated for C11H11O2, 175.1959) is in agreement with the molecular formula C11H10O2 corresponding to seven degrees of unsaturation. The IR spectrum showed absorption peaks indicating the presence of –OH (3,363 cm−1), Csp3-H (2,926 cm−1), and C=C (1,614 and 1,407 cm−1). Combined analysis of 1H and 13C NMR spectra of the isolated compound led to its identification as 8-methoxynaphthol (Fig. 2). 1H and 13C NMR data were compared to literature values of 8-methoxynaphthol isolated from the fungi Daldinia loculata[18] (Xylariaceae, Ascomycota, Table 1).
Figure 2.
Structure of 8-methoxynaphthol produced by the Annulohypoxylon stygium (PMEF05) from Pandanus simplex.
Table 1. Comparison of 1H and 13C NMR data with literature data.
Position 8-methoxynapthol, δH
measured at 400 MHz, CDCl38-methoxy-naphthol, δH
measured at 300 MHz, CDCl3[18]8-methoxynapthol, δc
measured at 100 MHz, CDCl38-methoxy-naphthol, δC
measured at 75 MHz, CDCl3[18]1 − − 154.7 154.6 2 7.33 (1H, m) 7.32 (1H, m) 118.9 118.0 3 7.36 (1H, m) 7.36 (1H, m) 125.6 125.8 4 7.42 (1H, d, J = 8.28 Hz) 7.44 (1H, m) 121.9 121.9 4a − − 136.9 136.9 5 7.29 (1H, dd, J = 7.80, 1.54 Hz) 7.29 (1H, m) 127.8 127.8 6 6.78 (1H, t, J = 7.80 Hz) 6.78 (1H, m) 110.5 110.6 7 6.88 (1H, dd, J = 7.80, 1.54 Hz) 6.93 (1H, m) 103.9 104.0 8 − − 156.3 156.3 8a − − 115.2 115.2 -CH3 4.06 (3H, s) 4.04 (3H, s) 56.2 56.2 -OH 9.35 (1H, s) 9.37 (1H, s) − − s : singlet; d: doublet; dd: doublet of a doublet; m: multiplet -
Several fungal endophytes have been previously reported from the tropical plant Pandanus. Fungal species belonging to the genera Colletotrichum, Chaetomium, Diaporthe, Glomerella, Guignardia, Lasiodiplodia, Lulworthia, Phoma, Phyllosticta, Trichoderma, and Truncatella were isolated from the leaves of P. amaryllifolius collected in the Philippines[13]. In another island ecosystem, the endemic P. rigidifolius found in Mauritius harbored novel species, Lepteutypa tropicalis[19], Ortanispora punctata[20], and some saprobic fungi ̶ Anthosthomella and Linocarpon[21]. Other species belonging to Astrocytis, Anthosthomella, and Pellucida were isolated from the endemic P. eydouxia, also from Mauritius[22]. Two other new species, Ascotaiwana mauritania and Niesslia pandanicola, were recorded from the endemic P. palustris[21]. In Thailand, P. odorifer hosted another novel species Hermatomyces krabiensis, H. pandanicola, and H. saikhuensis[23] including a novel genus, Anthostomelloides[24]. In this study, we isolated, identified, and reported Annulohypoxylon stygium for the first time as an endophyte of Pandanus simplex. This fungal species has been reported as endophytes of several host plants including the orchid Anoectochilus roxburghii[25], the red alga Bostrychia radicans[26], the red alga Asparagopsis taxiformis[27] and an unidentified host plant from China[28]. There are several factors including the morphological differences of the host plants, the environmental conditions, and other ecological variations that influence the assemblages of fungal endophytes in plants[29,30], and hence, could explain the varied host plants associated with our fungal endophyte.
Interestingly, fungal endophytes isolated from Pandanus also exhibited varied biological activities. For example, significant antimicrobial and antioxidant activities were reported from several endophytes associated with P. amaryllifolius[13]. Furthermore, novel secondary metabolites were identified from these fungal endophytes, e.g., a new macrolide (colletotriolide) from Colletotrichum sp.[14], diaportheones A and B from Diaporthe sp.[15], and guignardiol from Guignardia sp.[16] from the same host plant. Fungal endophytes also possess high antidiabetic potential[31]. For instance, six new diketopiperazine alkaloids with significant inhibition of the α-glucosidase enzyme were isolated from the endophytic fungus Aspergillus sp.[32]. The α-glucosidase enzyme plays an important role in carbohydrate digestion and absorption by catalyzing the conversion of polysaccharides into monosaccharides. Inhibitors of this enzyme could be used as initial treatment for patients with type 2 diabetes mellitus[33]. This is the primary motivation for this study. The crude and fractionated culture extracts of A. stygium displayed promising α-glucosidase inhibition activity, indicating the potential of this endophyte as a source of antidiabetic compounds.
Annulohypoxylon stygium has been previously reported for its bioactive secondary metabolites. For example, 16 compounds were isolated from A. stygium of which the compounds sterigmatocystin and palmarumycin CP2 exhibited selective cytotoxicty against cancer cells[25]. Compounds such as pyrogallol, (3R,4R)-3,4,5-trihydroxy-1-tetralone and tyrosol were also isolated from the same fungus from a red alga[27]. Furthermore, A. stygium isolated from an unidentified host plant produced a new compound annulostygilactone, along with nine known compounds[28]. In our study, we isolated and identified 8-methoxynaphthol from A. stygium living within Pandanus simplex.
The compound 8-methoxynaphthol is a polyketide and was recently reported from several endophytic fungi such as Alternaria sp. from the host Dasymachalon rostratum[34], from the mushroom Agaricus gennadii[35], and from the Diatrype palmicola[36]. Similarly, it was isolated from other fungal taxa[18, 37−44]. Moreover, 8-methoxynaphthol and another polyketide, 5-hydroxy-2-methylchromone, were regarded as chemotaxonomic markers of the genus Daldinia and other related fungal taxa[45]. The reports above suggest that 8-methoxynaphthol is a natural product of fungal origin.
With respect to its reported biological activities, 8-methoxynaphthol has no detectable antimicrobial activity[46] and a weak inhibition against Staphylococcus aureus, MRSA, and Microsporum gypseum[47]. However, the same compound displayed antagonistic activity against a plant pathogenic fungus[36], a good nematicidal activity[37], and an excellent radical scavenging activity[39]. Herein, the α-glucosidase inhibitory activity of 8-methoxynaphthol were presented with an IC50 of 676.3 ± 1.03 µg/mL, albeit the IC50 exhibited by the crude culture extracts was better (31.88 ± 2.86 µg/mL) than the pure compound. This differing degree of bioactivities is often observed with many natural fungal products. For example, the bioactivities exhibited by the crude extracts and its fractions or pure compounds which were isolated from cultures of endolichenic fungi and marine-derived fungi differed[48−50]. Perhaps the pool of different metabolites in the crude culture extracts acted synergistically leading to a better inhibitory activity.
-
Annulohypoxylon stygium is reported herein for the first time as a fungal endophyte of the Philippine endemic plant Pandanus simplex. The crude culture extract of A. stygium displayed better inhibition of the α-glucosidase enzyme than the fractionated and purified compounds. Further purification led to the isolation of the polyketide 8-methoxynaphthol, here also reported for the first time from A. stygium. This study shows the potential of endophytic fungi associated with tropical endemic plants to produce metabolites with antidiabetic potentials.
-
Pandanus simplex Merr. (Pandanaceae) is an endemic plant species in the Philippines that grows between 4 and 6 meters. The leaves are dark green and spirally crowded at the end of the branches, linear, elongated, with small, sharp spines on the margins. The trunk is cylindrical, with few branches on the upper part, and has prop roots near the base[51]. In this study, healthy leaves of P. simplex were collected at Luisiana (14°10'08.1" N, 121°30'28.0" E) in Laguna Province, Luzon Island, Philippines. The mature leaf samples were washed with tap water to remove adhering debris, air-dried, and then transferred to Ziploc bags and immediately transported to the laboratory for further processing. The identity of the host plant was verified by Danilo Tandang, National Museum of the Philippines (Authentication Control Number 1043).
The fungal endophyte, Annulohypoxylon stygium
-
For the isolation of fungal endophytes, leaf samples were cut into explants using a sterile puncher. Following surface-sterilization protocols[52], the explants were washed successively with 95% ethanol for 30 s, sterile distilled water for 30 s, commercial bleach:sterile distilled water (1:3) for 5 min, 95% ethanol for 30 s, and finally with sterile distilled water (four times, 30 s each). Surface-sterilized leaf explants were placed onto petri plates pre-filled with ½ strength malt extract agar (MEA) supplemented with 100 μg/mL Streptomycin and 400 μg/mL Benzylpenicillin to inhibit bacterial growth (30 leaf explants, 5 explants per plate). Fungi growing out of the leaf explants after 7 days were sub-cultured on freshly prepared full-strength MEA plates until pure cultures were obtained. From the preliminary bioactivity screening, one fungal endophyte (designated as PMEF05) showed excellent activity and thus was sent to Macrogen, Korea for molecular analysis. Genomic DNA from PMEF05 was extracted, amplified using the primer pairs ITS1 (TCCGTAGGTGAACCTGCGG) and ITS4 (TCCTCCGCTTATTGATATGC), and then subjected to DNA sequencing. The resulting sequence was initially edited and aligned using BioEdit Sequence Assembly Software for high sequence quality. After that, the aligned sequence of PMEF05 was uploaded to the GenBank database (Accession number: KY792891). Furthermore, the aligned sequence was uploaded in the nucleotide Basic Local Alignment Search Tool (BLAST, blast.ncbi.nlm.nih.gov) program. Species identification was determined from the lowest expected value (E-value) and the highest similarity percentage of the BLAST search output. Published related sequences, along with the sequence of PMEF05, were aligned and edited using MEGA ver. 5.05 (Molecular Evolutionary Genetic Analysis) via the accessory application ClustalW multiple alignment. A phylogenetic tree was constructed based on maximum likelihood (ML) analysis. The fungal endophyte Annulohypoxylon stygium is deposited at the UST Collection of Microbial Strains with accession number USTCMS4002.
Production and extraction of fungal culture extracts
-
An axenic culture of A. stygium was initially grown on Potato Dextrose Agar for 7 days. After incubation, one agar block (1 cm2) was inoculated on Erlenmeyer flask with 600 mL Potato dextrose broth (PDB, pH 7). After 4 weeks of incubation, 600 mL ethyl acetate was added to the culture broth, with the mycelial mass macerated and soaked overnight[53]. The organic layers were combined, dried over anhydrous Na2SO4, and concentrated in vacuo at < 40 °C to obtain the crude culture extract. The crude culture extract was stored at 4 °C until further processing.
Isolation and structure elucidation of 8-methoxynapthol
-
The crude culture extract of A. stygium was fractionated with a silica gel open column chromatography (70−230 mesh, Merck; height: 180 mm; internal diameter: 20 mm) using dichloromethane (DCM):acetone (0 to 50%) followed by acetone: methanol (50% to 0) at 10% increment gradient elution. Collected fractions were monitored using thin layer chromatography (TLC) in DCM:acetone (8:2) to obtain seven pooled fractions which were tested for their α-glucosidase activity thereafter. The first fraction, AsE-1 (45 mg), showed the highest inhibition and thus was further purified in silica gel open column chromatography (230−400 mesh, Merck; 78 mm height; 5 mm internal diameter) using hexane:DCM (50%), neat DCM and DCM:acetone (70%) solvents. Collected fractions were monitored using TLC in Hexane/DCM (1:1) to obtain a pure isolate, a light brown powder (30 mg; later identified as 8-methoxynapthol).
Several spectroscopic measurements were used to elucidate the structure of the isolated compound. Infrared radiation spectroscopy was carried out using IR Prestige 21 (Shimadzu, Japan) in KBr pellet sample preparation. Liquid chromatography-mass spectrometry (LCMS) was set up as follows: the isolated compound was initially dissolved in methanol to a concentration of 1 mg/mL and injected in 10 µL volume to 2695 HPLC Separation Module which is connected to a Micromass ZQ (Waters, USA). The column attached to the HPLC was a ZORBAX Eclipse XDB-C18 column (2.1 × 150 mm, 3.5 µm; Agilent, USA) while the mobile phase consisted of a gradient elution of solvent A (water with 0.02% formic acid) and solvent B (methanol with 0.02% formic acid starting with 95% A for 2.5 min then increasing to 100% B until 50 min and finally 100% B for 20 min. Electrospray Mass Spectrometry (ESI-MS) was carried out in positive ESI ionization mode with a cone voltage of 20V. The mass range was set from m/z 50−1,000 for ESI-MS range. For the nuclear magnetic resonance (NMR) experiments, the isolate was first dissolved in chloroform-d3 (CDCl3) and were recorded using Jeol ECS400 (JEOL, USA) at 400MHz and 100MHz field strengths for 1H and 13C nuclei, respectively. The obtained spectroscopic data were compared with published literature data.
In vitro α-glucosidase inhibition assay
-
The α-glucosidase inhibitory activity as a screening assay for antidiabetic activity of metabolites was carried out for the crude culture extracts and later with the fractionated and pure compounds. This was determined spectrophotometrically on a 96-well plate using α-glucosidase assay[54]. The assay mixture (160 μL) consisted of a mixture of 8 μL of test sample in DMSO, 112 μl phosphate buffer (pH 6.8), and 20 μL enzyme solution (0.2 Units/mL α-glucosidase in phosphate buffer) and incubated at 37 °C for 15 min. We also used 10% DMSO as a negative control and acarbose as a positive control. Then, 20 μL substrate solution (2.5 mM paranitrophenylglucopyranoside prepared in the same buffer) was added. The reaction was incubated at 37 °C for 15 min and stopped by adding 80 μL of 0.2 M Na2CO3 solution. Finally, the absorbance was measured at 405 nm. The inhibitory activity (%) was calculated as follows:
% inhibition = (1−(test sample − sample blankcontrol test − control blank))×100 where test sample = Absorbance of test sample + buffer + enzyme + substrate; Sample blank = Absorbance of test sample + buffer; Control test = absorbance of enzyme + buffer; Control blank = absorbance of buffer.
All reactions were carried out in three replications. The test concentrations used were 1,500, 100 and 10 µg/mL. Results were expressed as the average ± standard deviation of IC50 which were calculated by plotting a dose-response curve.
-
RRL Rondilla acknowledges the Accelerated Science and Technology Human Resource Development Program of the Department of Science and Technology - Science Education Institute (DOST-SEI) for the graduate scholarship grant. The authors would like to thank the University of Santo Tomas - Research Center for Natural and Applied Sciences for the laboratory facilities and additional financial support and the Kaohsiung Medical University - Graduate Institute of Natural Products for the use of equipment for spectroscopic analysis.
-
The authors declare that they have no conflict of interest.
- Copyright: © 2022 by the author(s). Published by Maximum Academic Press, Fayetteville, GA. This article is an open access article distributed under Creative Commons Attribution License (CC BY 4.0), visit https://creativecommons.org/licenses/by/4.0/.
-
About this article
Cite this article
Rondilla RRL, dela Cruz TEE, Chang F, Nonato MG. 2022. Annulohypoxylon stygium, a Pandanus simplex-associated fungal endophyte with α-glucosidase inhibitory activity. Studies in Fungi 7:4 doi: 10.48130/SIF-2022-0004
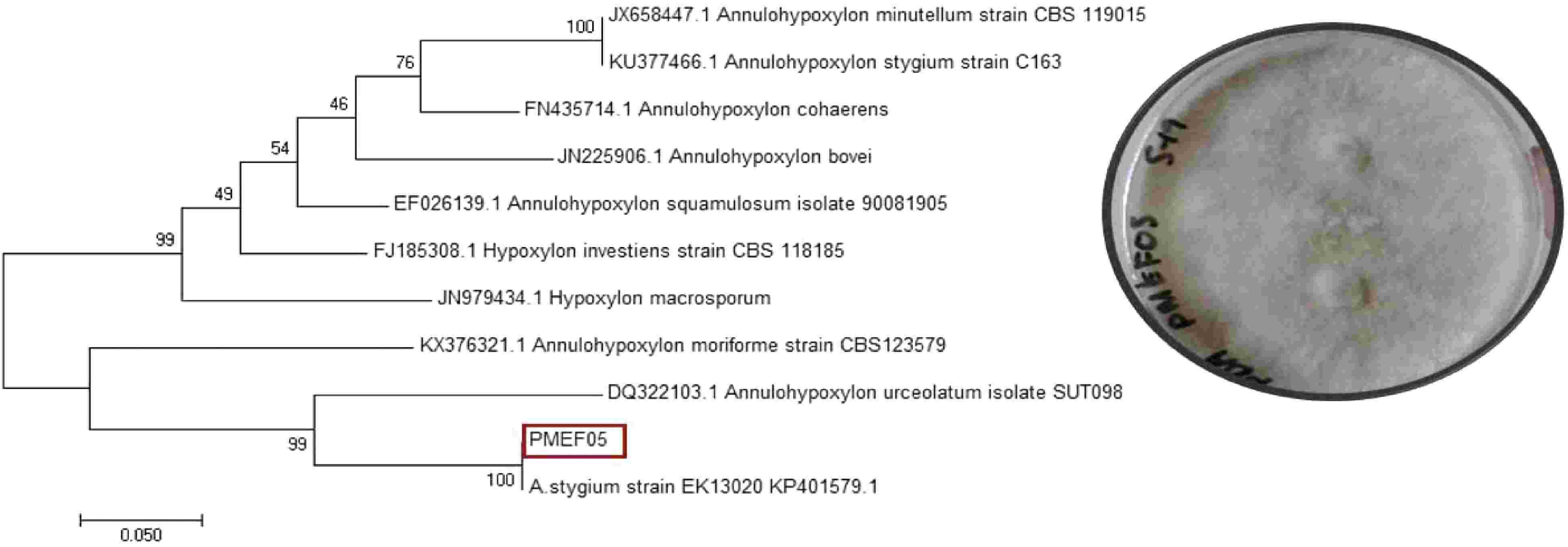