-
Industrial areas near arable land and coastal locations may engender a wide variety of environmental problems with impacts that extend considerably beyond the plant sites (Malizia et al., 2012; Muthusaravanan et al., 2018). These impacts range from relatively minor disturbances (such as temporary) to major disruptions e.g., water and soil pollution caused by toxic chemicals such as heavy metals (Tóth et al., 2016). The most commonly found heavy metals in industrial waste include arsenic, cadmium, chromium, copper, lead, nickel and zinc (Papamanolis et al., 2018) and are the most frequent form of environmental pollution around industrial areas (Malizia et al., 2012). Previously studies indicated that the interaction between Pb (II) ions, organic matter (humic acids) (Giannakopoulos et al., 2005) and clays (Giannakopoulos et al., 2006) results in the formation and stabilization of new radicals with a striking feature being their unusually low g-values, i.e., below the free electron g-value allowing for the formation of stable chelating Pb (II) complexes of Pb (II) with humic substances of soil. This metal-accumulation in soils at concentrations above the upper limit may cause soil malfunctions and toxicity in plants, animals and humans (Tóth et al., 2016; MEF, 2007). In the case of plants, the heavy metal uptake is evaluated by the bioaccumulation factor (BF) (Zhuang et al., 2007). As reported by Netty et al. (2013), if BF > 1, the plants are defined as 'metal super' accumulators; when the BF ranges between 0.1 and 1 the plants belong to the 'moderate' accumulators; and when the BF is between 0.01 and 0.1 the plants are classified as 'weak' accumulators.
According to the principles of sustainable development in order to preserve natural resources and minimise the environmental impact of pollution upon plants, animals and humans, every agricultural production in multi-metal contaminated soils must be evaluated and provide an assessment and recommendations on the necessary actions required for crop sustainability (Tripathi et al., 2017). Nowadays, natural zeolites have been increasingly used in various application areas such as industry (Mench et al., 2002), agriculture (Tsadilas et al., 1997), environmental protection, and even medicine (Eroglu et al., 2017). More than 40 naturally occurring zeolites were reported by different research groups, and clinoptilolite, erionite, chabazite, heulandite, mordenite, stilbit and philipsite are the most well-known (Eroglu et al., 2017; Polat et al., 2004). Natural zeolites were discovered more than 200 years ago, and are studied due to their peculiar characteristics such as high degree of hydration, high degree of crystallinity, low density and the large volume of free spaces (dehydrated); shape selectivity, ion exchange characteristics, catalytic characteristics, sorption of molecules and ions and electric conductivity (Morante-Carballo et al., 2021). They can be described as 'crystalline aluminosilicates with a three-dimensional structure based on repeating units of silicon-oxygen (SiO4) and aluminium-oxygen (AlO4) tetrahedra', and are also attributed as 'molecular sieves' due to their ability to accept and reject molecules based on their size (Morante-Carballo et al., 2021). The studies mainly focus on water treatment, wastewater management, soil decontamination, and nuclear waste. Zeolite applications in environmental remediation are mainly due to its ion exchange properties, where, depending on the type of zeolite, its pore size, the shape of its internal channels, and the type of exchange cation—it can interact with cations from the environment, with which it can have high selectivity such as heavy metals (Morante-Carballo et al., 2021).
The most common for agricultural applications is clinoptilolite since it has high absorption, cation exchange, catalysis and dehydration capacities (Polat et al., 2004). Its ability to absorb toxic and radioactive ions from liquids and soils is of great importance to ecology and human health (Misaelides et al., 2011). Kim et al. (2012), indicated that the zeolite demonstrate high heavy metal immobilization efficiency, reduces the availability of plants, and simultaneously mitigates the threat of food chain contamination with heavy metal ions. In particular, the addition of zeolite (at concentration < 1%) in the metal-contaminated soils contributes significantly to plant growth by limiting the availability of heavy metals (Muthusaravanan et al., 2018). As reported by Ahmed et al. (2010), and Prasad et al. (2014), the presence of zeolite in a metal contaminated soil increases the soil surface due to its porous structure and results in better aeration of the plants. Since zeolite absorbs heavy metals and nutrients enables the increase of plant growth and crop yield.
A survey report issued by the Greek Ministry of Environment on the rehabilitation of sites contaminated by industrial and hazardous waste showed that there are 2,000 contaminated sites in Greece, out of which 300 need immediate rehabilitation according to the principles of sustainable development in order to preserve natural resources and minimise the environmental impact of pollution on future generations (GME, 2016).
The purpose of this investigation was to examine the alternative use of white clover as a metal accumulator plant and as a raw material (feed) after its cultivation in heavy-metal contaminated soils. The selection of white clover is due to its properties as a versatile plant species that is widely used in sustainable agriculture to improve soil fertility (Abbasi & Khan, 2004) and has great agronomic value as it is used as forage, cover crop and a basic feed (Bailey & Laidlaw, 1998). In addition, the possibility of using zeolite as a strengthening agent for the restoration of pollution and the immobilization of heavy metals was assessed. The aforementioned characteristics are in accordance with the new EU Common Agricultural Policy (Green Deal) (EC, 2020). Nowadays, the EU Farm to Fork strategy has increased the urgency to focus research on the use of white clover for reduction of chemical N fertiliser use and the restoration of pollution and the immobilization of heavy metals (EC, 2020a). In this framework, the evaluation of the effects of heavy metals on crop growth in multi-metal contaminated soils is necessary for reducing the associated risks, making the land resources available for agricultural production, enhancing food security and scaling down land tenure problems arising from changes in the land use pattern (EC, 2020a; Tripathi et al., 2017).
-
Soil sampling was applied according to the protocol developed by Ahmad et al. (2018), and carried out on two field sites in the Thessaly Region of Greece. The first experimental site (coded as polluted soil, 'P') was located on soil polluted with heavy metals (Pb, Cd and Zn) in Volos, Greece (GME, 2016). In particular, the 'P' site is agricultural land affected by heavy metals soil contamination resulting from a decommissioned industrial activity. This soil has contents of Cd, Pb and Zn that exceed the limits set by Greek law for arable lands (GME, 2015) and on the basis of the GME's survey (GME, 2016) needs immediate rehabilitation according to the principles of sustainable development (Tripathi et al., 2017). The second experimental site (coded as unpolluted soil, 'C') was located on unpolluted land (control) surrounding the Institute of Industrial and Forage crops of the Hellenic Agricultural Organization-Demeter (HAO-DEMETER) at Larissa, Greece.
Soil sample preparation
-
Soil samples were collected, according to the protocol developed by Ahmad et al. (2018), from the outer surface, i.e., 5 to 30 cm depth, after removing surface contamination that may consist of leaves or other transportable materials using a soil sampler or a simple shovel, up to the depth of 30 cm. The samples were collected in self-locking polythene bags and were sealed in double bags. The samples were then mixed and dried at 30 °C for 48 h, then ground with a porcelain mortar and pestle and sieved through a 2 mm sieve to remove large debris, stones and pebbles. The < 2 mm fraction of the soil was used for all soil analyses.
Soil analysis
-
Soil physicochemical analysis was performed according to the protocol developed by Allison (1965), and Allison & Moodie (1965), whilst the essential nutrients such as Nitrogen, Phosphorus, and Potassium were determined as described by Hutchinson & Meema (1987). Particle-size analysis was performed by the hydrometer method (Bouyoucos et al., 1962), pH and electrical conductivity (EC) were measured in 1:1 suspension with water, and organic carbon was determined by the wet oxidation method (Walkley & Black, 1934). The method used for carbonate content was the Bernard method, by measuring the evolved CO2 after the addition of HCl (Nelson, 1982). Soil-available P was extracted by employing the method suggested by Olsen & Sommers (1982) and determined using spectrophotometry. Exchangeable cations were determined after extraction with 1M CH3COONH4 at pH 7 (Knudsen et al., 1982), with a Jenway PFP7 flame photometer used for measuring K (Jones & Case, 1990).
Moreover, the total metal (Cd, Pb, and Zn) concentrations were determined by an atomic absorption spectrophotometer (Thermo Scientific, iCE 3000 Series) according to Hutchinson and Meema's protocol (Hutchinson & Meema, 1987) as described in the EPA 3050 method (determination of pseudo-total heavy metals in soil samples by aqua regia digestion at 140 oC for 5 h with 3:1 concentrated HCl : HNO3) (ISO/DIS 11466, 1994). Pseudo-total metals in particular, are acid-leachable metals that are not part of the silicate matrix (Relic et al., 2011). All the results are summarised in Table 1. X-ray diffraction (XRD) was performed on the soil samples 'C' and 'P' to assess the altered degree of mineralogical composition in the soils after amendments were added (1% Zeolite), in order to decipher the soil-texture of the modified soils as described earlier (Michas et al., 2020). Powder X-ray diffraction (XRD) of the soils was performed on a Bruker D8 Advance diffractometer, using a nickel-filtered CuKα (1.5418 Å) radiation source. The data collection was carried out in the range of 5° ≤ 2θ ≤ 80° with a step of 0.02° and an acquisition time of 0.5 s and diffraction data were analyzed by the Bruker DIFFRAC.EVA software, using the fully Crystallography Open Database, as described earlier (Flogeac et al., 2005).
Table 1. Nutrients, physical and chemical characteristics of the tested soils.
Soil Nutrients and physicochemical properties Metal concentration (mg/kg dry soil) N
(g/100g)P
(mg/kg)K
(cmol+/kg)pH
(+0.01)Organic
matter
%CaCO3 % CEC
(meq/100 g
dry soil)Sand
%Clay
%Silt
%Soil
typeCd Pb Zn 'P' 0.10 11 0.74 8.24 3.21 1.10 34.92 64.0 12.1 24.2 Sandy loam 4.30a 291.10a 1,458.12a 'C' 0.11 10 1.51 7.32 0.67 2.21 36.31 31.0 44.2 25.0 Clay nd** 17.32c 76.21c Maximum allowable values* 1.00 60.0 200.0 * Ministry of the Environment–Finland (MEF, 2007). nd**: Not Detectable. P: polluted soil, C: unpolluted soil (control). Different letters in each column indicate statistically significant differences (F-test, p < 0.05). Natural zeolite (Clinoptilolite)
-
Clinoptilolite rich tuff Zeolite (commercial sample: BZM 0.15, supplied by Imerys Minerals AD, Bulgaria) was used in this work is from the Beli Plast deposit (Bulgaria) and contains nearly 85 wt. % clinoptilolite, opal-CT ~15 wt. % and cation exchange capacity (CEC) > 150 cmol+/kg. The chemical composition (wt. %) – 69.62 SiO2, 13.62 Al2O3, 2.94 K2O, 0.55 Na2O, 0.75 Fe2O3, 0.11 TiO2, 3.28 CaO and 0.9 MnO of this clinoptilolite results from the following crystal chemical formula: Na0.3K1.8Ca2.0Mg0.3Al6.3Si28.7O72.21H2O (Lihareva et al., 2015).
Pot culture experiments
-
In order to investigate the soil remediation potential of zeolite we applied 1% zeolite in a heavy-metal polluted Greek soil cultivated with white clover and in a non-polluted control soil. The addition of amendments into the soil could make heavy metals less bioavailable and thus could be suitable for heavy metal uptake mitigation by plants (Ahmed et al., 2010; Prasad et al., 2014). However, information about the effect of inorganic amendments on agricultural soils mainly with Cd (metal with high toxicity degree) is scarce. Keller et al. (2005) reported that the addition of zeolite in heavy metal-polluted soil at a dose of 1%, instead of 5%, was the most efficient way for reducing the average Cd concentration in tobacco plants. Under these considerations, pot experiments were performed in a HAO-DEMETER greenhouse to investigate the bio-accumulation capacity of White clover in a Cd, Pb, and Zn polluted soil remediation program in conjunction with incorporating 1% zeolite into the soil, as described earlier (Michas et al., 2020).
Briefly, an equal number of pots were filled with 3 kg of contaminated, soil 'P' (12 pots) and non-contaminated, soil 'C' (12 pots). For each soil type half of the pots (6 pots in each soil) were treated with 1% zeolite per kg as descripted by Keller et al. (2005). Twenty seeds of white clover per pot were sown for each soil treatment while non-sown pots per treatment were left as a control. Three replicates were applied that correspond to 24 pots in total. Across all the treatments, 1.1 g P was applied to pots sown with white clover (Pederson, 1995) after taking into account the soil content of the above nutrients based on Table 1. The protocol proposed by Li et al. (2018), was followed during the growth period whereby all the pots remained free from weeds. The moisture content of each pot was adjusted to 60% of soil water capacity (Li et al., 2018) by weighing the pots twice a week and supplementing the corresponding water quantity. For the water capacity calculation, the weight of dry soil in each pot was calculated and subsequently water was added until saturation. The pot was weighed again and the difference corresponded to the water capacity value. Total plant samples were collected 90 d after sowing. Samples were washed with tap water to eliminate soil particles, rinsed with 0.1 M HCl and 0.1% soap substitute and deionized water, dried at 75 °C for 24 h and the dry matter was finally measured.
Quantitative analysis of metal uptake by plants: bioaccumulation factor (BF)
-
The BF in white clover plants with Cd, Pb, and Zn was calculated with the following equation (1) (Zhuang et al., 2007):
(1) where: Cpt is the metal concentration in plant tissue, and Cs is the metal concentration in soil.
Specifically, Cpt corresponds to Cd, Pb, and Zn concentration in roots and shoots, whereas Ctot corresponds to the total heavy metal concentration in the soil types used for the experiment. The total Cd, Pb and Zn concentrations in plant tissues were determined by Dry Ashing Procedures (Jones & Case, 1990), whilst the available concentrations of Pb, Cd and Zn in the soil were determined with the extraction method (Giannakopoulos et al., 2017). For the statistical analysis a JPM8 statistical program (SAS Institute, ver. 2nd, 2009) was used to analyze the variance and compare the means. All differences among all soil samples were determined with One-way Anova (F-test, p < 0.05).
-
The composition of the 'C' soil samples that were collected from around the HAO-DEMETER institute was found to be clayey (USDA, 2009) (Table 1); the samples were described as neutral, pH = 7.32, with very low organic matter, 0.67%, and a high ion exchange capacity 36.31 meq/100gr. On the other hand, the 'P' soil samples near the industrial area were moderately alkaline and rich in organic matter (3.21%) (Table 1).
The CEC was high, 34.92 meq/100gr, resulting in greater sorption and immobilization of the metals (Lasat, 2000). The soil was sandy loamy belonging to the coarse soils (USDA, 2009) with high pH (Table 1). The XRD diffractograms of the soil 'P' and soil 'C' in Fig. 1 show major peaks of SiO2 (Flogeac et al., 2005).
Figure 1.
X-ray powder diffraction pattern of the (I) soil-'C' and (II) soil-'P'. The dotted lines show similar peaks. (C1: unpolluted soil, C2: unpolluted soil with the addition of 1% zeolite, P1: polluted soil and P2: polluted soil with the addition of 1% zeolite).
Table 1 summarizes the concentrations of heavy metals Cd, Pb, and Zn in the soils used as plant substrates (not detectable, 17.32 and 76.21 mg/kg of dry soil C and 4.3, 291.1 and 1,458.12 mg/kg of dry soil P, in Cd, Pb, and Zn, respectively). According to our findings, the concentrations of Cd, Pb, and Zn in the contaminated soil 'P' were higher than the maximum allowable values of MEF (2007) (1, 60 and 200 mg/kg of dry soil, in Cd, Pb, and Zn, respectively).
Dry matter measurements of white clover plants
-
White clover plants grown in contaminated soil 'P' and unpolluted 'C' soil, yielded higher dry matter of 2.83 g (= 8.33 − 5.50) and of 1.6 g (= 6.83 − 5.17) respectively, when the soil was amended with zeolite (Fig. 2). It is noteworthy that the largest quantity of biomass for White clover plants has been observed in contaminated 'P2' soil in the presence of zeolite. In particular, the addition of zeolite in 'P' and 'C' soils increased the dry biomass of plants by 51.4% and 32.1%, respectively.
Figure 2.
Dry weight of white clover plants grown on the different tested soils. (P1: polluted soil without the addition of 1% zeolite, P2: polluted soil with the addition of 1% zeolite, C1: unpolluted soil without the addition of 1% zeolite, C2: unpolluted soil with the addition of 1% zeolite). * Different letters in each column indicate statistically significant differences (F-test, p < 0.05). Values ± SD.
Concentration of Cd, Pb and Zn on the total plant tissue (roots and shoots) of white clover plants
-
The heavy metal bioaccumulation order in the total plant tissues of white clover plant followed the sequence: Zn > Pb > Cd. The concentrations of Cd, Pb, and Zn were also found to be significantly higher in the total plant tissue of white clover grown in contaminated soil, with 1.93, 11.74 and 199.31 mg/kg respectively, compared to that measured in the total plant tissue in unpolluted soil (nd**), 5.58 and 60.92 mg/kg, respectively (Table 2).
Table 2. Concentration of Cd, Pb and Zn in the total plant tissue of white clover plants.
Soil White clover (mg/kg dry weight of plant) Cd Pb Zn P1 1.93a* 11.74a 199.31a P2 1.43b 7.98b 141.95b C1 nd** 5.58c 60.92c C2 nd** 3.66d 54.16c Normal consumption
limits as dry biomass:0.50 30.00 300.00 P1: polluted soil without the addition of 1% zeolite, P2: polluted soil with the addition of 1% zeolite, C1: unpolluted soil without the addition of 1% zeolite, C2: unpolluted soil with the addition of 1% zeolite. *Different letters in each column indicate statistically significant differences (F-test, p < 0.05) nd**: not detectable. The statistical significant differences were also detected in the concentration of Cd, Pb and Zn in the total plant tissues of white clover plants grown in contaminated soil with or without zeolite (Table 2). The treatments of white clover grown in soil P with zeolite showed a significant 28.8 % reduction in Zn uptake, 25.9 % reduction in Cd uptake and 32 % reduction in Pb uptake. The reduction of Pb uptake of white clover in soil C after the zeolite amendment was significant, reaching 34.4%.
On the basis of Table 2, it is noteworthy that the concentration of Cd in the white clover plant tissue developed in contaminated soil with or without zeolite in soil was higher, namely 1.43, 1.93 mg/kg dry weight of plant respectively, than normal consumption limits as dry biomass, namely 0.50 mg/kg dry weight of plant.
BF of white clover plants
-
White clover was found to act as a 'moderate' accumulator of Cd and Zn, whilst the ability of white clover to accumulate Pb seems to be 'weak' (Fig. 3). The addition of zeolite to the soil contributed to the reduction in the BF of heavy metals (Fig. 3).
Figure 3.
Bioaccumulation factor (BF) of white clover for Cd, Pb, and Zn. (P1: polluted soil without the addition of 1% zeolite, and P2: polluted soil with the addition of 1% zeolite,). Different letters in each column indicate statistically significant differences (F-test, p < 0.05). Values ± SD.
Determination of heavy metals and their availability to plants in soils after harvesting white clover plants
-
A decrease in the total concentration of Cd (45.81%), Pb (25.91%), and Zn (31.64%) was observed in the polluted soil 'P1' without the addition of 1% zeolite (Tables 1 & 3) after the cultivation of white clover. This decrease, in case of the polluted soil 'P2' with the addition of 1% zeolite was lower, namely: 39.01 % for Cd, 24.31% for Pb, and 28.45% for Zn (Table 3). Also, there is a statistically significant difference between the total and available heavy metals' soil concentration in polluted and unpolluted soil, studied after the plants harvest (Table 3). The potentially available metal fraction in soils may be a strong indication of recent metal depositions. Thus, using both total and potential available data sets for metal forms and examining their interrelations may assist with comprehending the possible effects by metals levels in soils on biological systems and the sources of current pollution events (Massas et al., 2013; Michas et al., 2020). However, after the white clover plants harvest, the total and available metal concentrations in the soil followed the descending order: Cd > Zn > Pb, independently of the treatment used with or without zeolite (Table 3).
Table 3. Total and available metal concentration in the soils after harvesting white clover plants.
Soil Metal concentration (mg/kg dry soil) Total Available Cd Pb Zn Cd Pb Zn P1 2.33a 215.67a* 996.67a 0.39a 31.00a 84.67a P2 2.74b 220.33b 1043.33b 0.40a 32.33a 93.67b C1 nd** 12.00c 73.33c nd** 2.07b 5.97c C2 nd** 13.33c 70.00c nd** 2.1b 6.87c P1: polluted soil without the addition of 1% zeolite. P2: polluted soil with the addition of 1% zeolite. C1: unpolluted soil without the addition of 1% zeolite. C2: unpolluted soil with the addition of 1% zeolite. * Different letters in each column indicate statistically significant differences (F-test, p < 0.05). -
The low percentage addition of zeolite in soil (Michas et al., 2020) did not alter the soil matrix as was found by the XRD diffractograms (Fig. 1). On the other hand the polluted soils where rich in organic matter (Table 1), as compared to the average content of organic matter in Greek soils, which ranges between 1-2.5% (Zdruli et al., 2004).
To assess the level of heavy metals concentration in the soil, we consulted the maximum allowable limits of heavy metals in the soil that was adopted by the Finnish legislation (MEF, 2007) and after investigation between the many different options of European countries (EC) (Tóth et al., 2016). As mentioned by Carlon et al. (2007), the above metal concentration limits of MEF (2007), taken into account herein, represent the average values in the national laws of different European Union countries, and are internationally implemented for evaluating and utilizing polluted soils in agricultural production (UNEP, 2013).
Hence the studied soil was heavily polluted with heavy metals (Table 1), indicating that the industrial zone significantly contributes to the contamination of the adjacent areas with Cd, Pb and Zn (MEF, 2007). In addition, as reported by Iqbal & Saeed (2007) and by Vijayaraghavan & Yun (2008), in alkaline soils with pH > 8, the available concentration decreases due to precipitation and hydrolysis of the metal complexes, while some heavy metals were combined with carbonated ions by replacing calcium in the crystalline matrix of the carbonated minerals, thus limiting their availability (Hooda, 2010).
The application of zeolite in both control and polluted soils resulted in the increase of white clover dry biomass (Fig. 2). These results are in accordance with Lin & Zhou (2009), whereby the incorporation of zeolite into soil contaminated with Pb, Cd and Cu has a positive effect on plant growth by preventing the uptake of heavy metals. As reported by Bidar et al. (2007), this could be attributed to the physiological resistance mechanisms with less oxidative damage in white clover in comparison to other plants. Plants exposed to environmental stress (i.e. temperature changes, UV light, ozone exposure, water deficiency, metallic ion excess, presence of redox active heavy metals) generally showed some alterations in the electron transport processes such as photosynthesis (chloroplast) and mitochondrial respiration. Thereafter, the disruption in the electron transport contributes to Reactive Oxygen Species (ROS) production, as well as the impairment in sophisticated enzymatic and/or non-enzymatic ROS scavenging systems. To counteract the adverse effects of ROS, plants developed antioxidant defense systems comprising enzymes as catalases, peroxidases superoxide dismutases and non-enzymatic constituents (a-tocopherol, ascorbate, reduced glutathione, etc.), which remove, neutralize, and/or scavenge oxidative species (Bidar et al., 2007).
As expected, the total plant tissue of white clover in the polluted soil was found to possess significant higher values of heavy metals (> 110%) than in the control soil (Table 2), which are in accordance with Rebah et al. (2002), and Khan et al. (2011). Moreover, the application of zeolite resulted in the significant reduction of the heavy metals uptake of white clover in the polluted soil (Table 2), that is in line with Chlopecka & Andriano (1997), which found that zeolite and apatite can reduce the Cd, Pb and Zn accumulation in maize leaves. In fact, zeolite with its negative charge provides an ideal trap for positive cations such as Na+, Ca2+, Mg2+ and K+, and for positively charged groups such as water and NH4+ (Taffarel & Rubio, 2009). Therefore carbonate and nitrate ions can be bound within zeolites. Inasmuch, metallic cations are attracted in the same way and water can be absorbed by zeolites (Rhoades, 1982). Absorbed cations due to their weak attraction are relatively mobile and can be replaced by using ion-exchange techniques. In addition, as reported by Kim et al. (2012), zeolites have a wide range of surface areas containing multi-functional groups where heavy metals can be adsorbed and complexed.
Nevertheless, since for the case of Cd the application of zeolite did not result in the reduction of its content in soil in lower levels than 0.5 mg/kg (Table 2), this signifies that the consumption of its dry mass from animals such as cattle, sheep, pigs and chickens could cause serious toxic effects and even lead to death. Potentially, if the concentration of heavy metals in roots and shoots had been measured separately, the results could be more encouraging with regard to its consumption by animals. This is confirmed by studies of Bidar et al. (2007), and Farrag et al. (2016), according to which the roots of white clover plants store higher concentrations of Cd, Pb and Zn than those measured in the shoots (stem) and leaves of the plant. As reported by Farrag et al. (2016), this limitation can be overcome by the removal of the roots of white clover plants from biomass (animal feed), resulting in low risk to animals (NRC, 1980).
In this point it is important to state that even if the ability of white clover to accumulate Pb seems to be 'weak', the application of zeolite resulted in 32%–34.4% reduction in both polluted and control soil (Table 2). Therefore, the synchronous application of zeolite and white clover could result as a good practice for soil rehabilitation.
Our findings are in accordance with Pricop et al. (2010), regarding the bioaccumulation capacity of white clover in heavy metal contaminated soils. Additionally, Peuke & Rennenberg (2005) found that constructive and cost-effective phytoremediation requires the use of plants with a bioaccumulation coefficient over 10 and biomass production capacity of at least 20 tn/ha, or alternatively the plants must at least have a bioaccumulation coefficient of 20 and biomass production greater than 10 tn/ha. On the other hand, the investigation of Ali et al. (2012), showed that the use of white clover alexandrinum for phytoremediation (by considering uprooting the plants in which case the accumulated heavy metals in the roots are removed from the soil) has many advantages. For example, it produces considerable biomass, has a relatively short life cycle, is resistant to prevailing environmental and climatic conditions and above all offers multiple harvests in a single growth period. Thus, this candidate species can be used for phytoremediation of toxic heavy metals (Michas et al., 2020).
According to Massas et al. (2013), using both total and potential available data sets for metal forms and examining their interrelations may assist with comprehending the possible effects by metals levels in soils on biological systems and the sources of current pollution events (Table 3). In this context we found that the addition of zeolite soil controls the heavy-metal pollution in the soil matrix (Table 3), not allowing the white clover plants to bio-accumulate them into their biomass (Table 2). According to Ahmed et al. (2010), the presence of zeolite in the soil increases the retention of heavy metals as an absorber, thus, increasing crop yield. In fact, Ali et al. (2012), indicated that the white clover species such as Trifolium alexandrinum can be used for phytoremediation of toxic heavy metals and metalloids, including Cd, Pb, and Zn (Gatliff et al., 2016). Nevertheless, the total concentration of metals in the soil after the cultivation of white clover plants is lower but still above the permitted limits (Table 3) for Cd, Pb, and Zn (MEF, 2007), so perhaps the systematic monoculture of white clover with or without the zeolite amendment in the future may define whether the time and energy cost are enough to justify the practice.
-
This study demonstrated that the white clover plant is a 'moderate' accumulator of Cd and Zn, while its ability to accumulate Pb is 'weak'; the addition of zeolite in the heavy metal contaminated soils can minimise metal pollution in the environment and positively contribute to the growth of white clover plants. Moreover, the study showed that the concentration of Cd in the white clover plant tissue developed in contaminated soil with or without zeolite in soil was higher in its dry biomass than the normal consumption limits. Therefore, the white clover dry biomass consumption from animals such as cattle, sheep, pigs and chickens could cause serious bioaccumulation toxic effects and even lead to death. Consequently, the white clover alone seems to be inappropriate for soil remediation from Cd contamination in the context of sustainable development. On the other hand, the concentrations of Pb and Zn in the total plant tissue of white clover plants are below the maximum allowable limits for animal feed, resulting in low risk to animals. In order to achieve this goal contaminated fields with high concentrations of heavy metals should not be left unexploited during any growing season in order to decontaminate or reduce their pollution (exploitation of contaminated fields). Furthermore, previously polluted fields can be decontaminated or reduce their pollution with white clover which can be promoted for other uses such as dry fodder or for energy production contributing to the financial support of farmers. Moreover, the reduction of the soil heavy metals concentration leads to a reduction of the toxic elements amount that enter the underground aquifer with direct positive effects on the agro-ecosystem and the environment in general. Finally, the application of zeolite contributes to the increase of agricultural production as well as to the production of safe agricultural products. These results are expected to increase the productive actors' awareness on environmental issues and particularly on those related to the preservation of soil quality. In fact since soil is a natural resource closely related to agricultural productivity it is our outmost duty to protect it in order to provide sustainability for the future generations.
Preliminary results of the project were presented at the 11th European Conference on Pesticides and Related Organic Micropollutants & 17th Symposium on Chemistry and Fate of Modern Pesticides in Ioannina, Greece 23 to 26 June 2022. A part of the research project was funded by the University of Patras, in the frame work of the program 'MEDIKOS, 81938'. Also, we would like to thank Institute of Industrial and Forage crops HAO-DEMETER in Larissa, Greece for the logistic infrastructure that was provided in the experiments.
-
Marios Drosos is the Editorial Board member of Journal Soil Science and Envrionment. He was blinded from reviewing or making decisions on the manuscript. The article was subject to the journal's standard procedures, with peer-review handled independently of this Editorial Board members and his research groups.
-
# These authors contributed equally: Vasilios Sotiriou, Georgios Michas
- Copyright: © 2023 by the author(s). Published by Maximum Academic Press, Fayetteville, GA. This article is an open access article distributed under Creative Commons Attribution License (CC BY 4.0), visit https://creativecommons.org/licenses/by/4.0/.
-
About this article
Cite this article
Sotiriou V, Michas G, Xiong L, Drosos M, Vlachostergios D, et al. 2023. Effects of heavy metal ions on white clover (Trifolium repens L.) growth in Cd, Pb and Zn contaminated soils using zeolite. Soil Science and Environment 2:4 doi: 10.48130/SSE-2023-0004
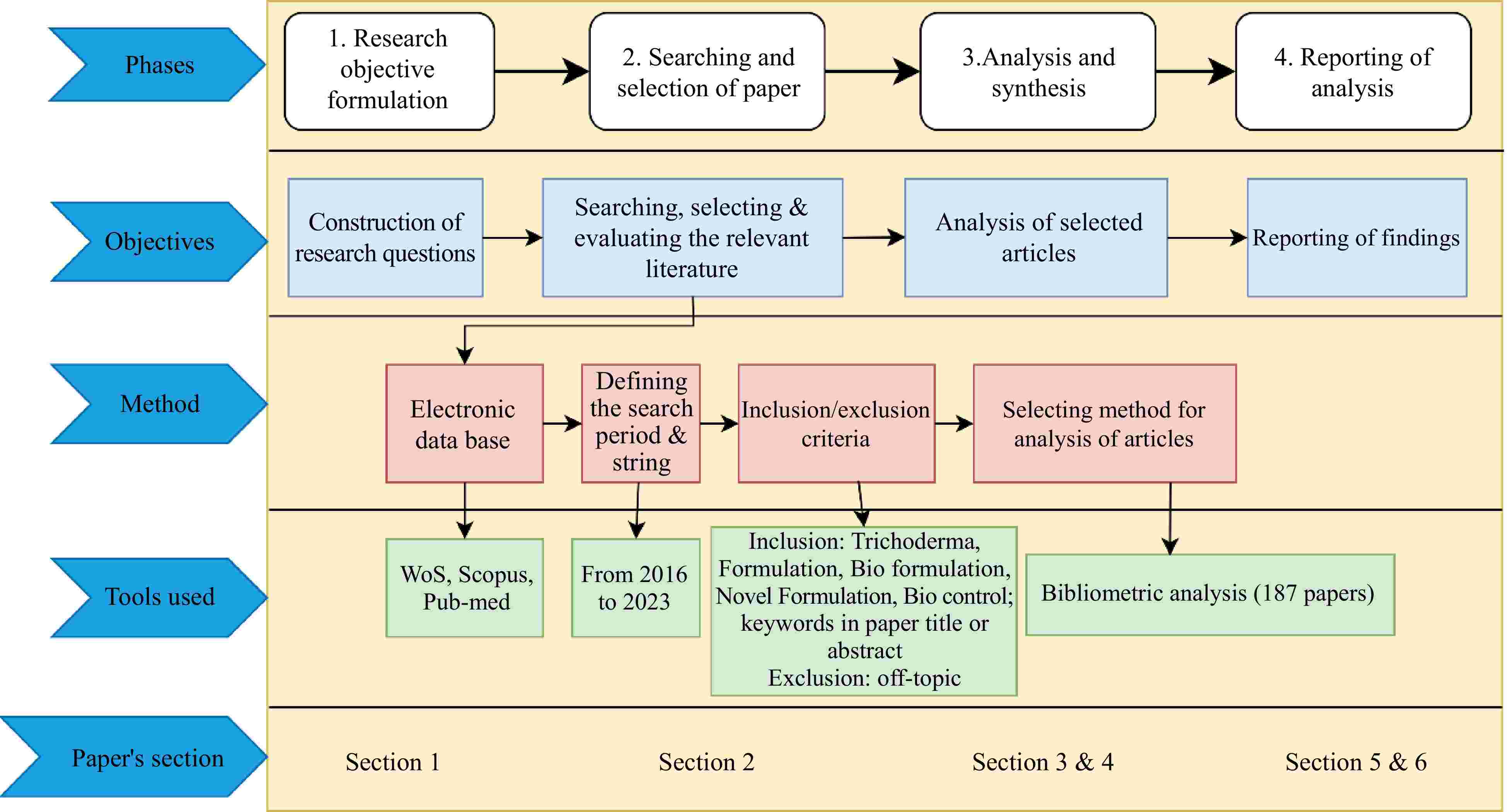