-
Oxalis triangularis 'Purpurea', a perennial herb belonging to Oxalidaceae is native to South America. It has palmately compound leaves, containing three inverted triangular leaflets with entire margins. The leaves are purplish red and cluster at the base[1,2]. O. triangularis 'Purpurea' is widely cultivated as a foliage potted flower, flower bed border plants, and garden ground cover ornamental plants. It is also a herbaceous plant with antibacterial, antifungal, and anticancer potential[3]. Its flowers show the characteristic of heterostyly and self-incompatibility. It cannot produce seeds by self-crossing but can only reproduce asexually by subterranean bulbs[4]. Therefore, it is difficult to obtain excellent varieties quickly by conventional breeding. Transgenic technology is an effective alternative to modifying horticultural flower traits by introducing other good trait genes. It is also an effective way to accelerate the genetic improvement of garden flowers[5]. However, the research on the genetic transformation of O. triangularis 'Purpurea' is still in the preliminary stage. It is far from satisfying the requirements of genetic improvement technology and commercial production.
In vitro culture is conducive to expanding production scale, improving plant quality, promoting germplasm improvement, and providing stable plant materials for genetic transformation and gene editing technologies[6]. For the breeding of new varieties, the tissue culture of plants makes it easier to separate mutations[7]. There are still problems in studying rapid propagation of O. triangularis 'Purpurea' in vitro. For example, the callus induction rate and the number of induced cluster buds are low. The growth rate is also slow. Callus is an ideal transgenic material. It has the advantages of stable proliferation, high regeneration rate, and agrobacterium resistance[8]. At present, studies on in vitro culture of Asparagus officinalis[9], Dendrobium wilsonii[10], Zingiber officinale[11] and other plants have been reported. There have been studies on the in vitro flowering technology of Oxalis L. Prasuna et al. used Oxalis corniculata as material for tissue culture in vitro, and found that plant growth regulators, sucrose, MS, and light conditions could transform plants from vegetative growth to reproductive growth[12]. In addition to commonly used nutrients, the addition of TDZ to the medium can induce the proliferation of plant shoots in tissue culture[13−15]. However, there are few studies on embryonic callus of O. triangularis 'Purpurea', and the specific culture method is still unclear.
To explore the culture scheme of O. triangularis 'Purpurea' in vitro, the petiole and leaf of the plant were used as explants. The basic medium with 6-BA and NAA was supplemented with additional TDZ in different concentrations for callus induction, proliferation, adventitious bud rooting, and bulb induction. An in vitro regeneration system of O. triangularis 'Purpurea' can be established to promote the development of transgenic plants. Meanwhile, it provides a basis for gene function study and has important reference significance for the induction of embryonic callus and genetic engineering breeding of other plants belonging to Oxalis.
-
In the medium containing NAA and 6-BA, using petioles as explants could effectively improve the culture efficiency of the sterile plants of O. triangularis 'Purpurea'[16]. The study selected the tender petioles of O. triangularis 'Purpurea' with good greenhouse growth status as experimental materials. Petioles were rinsed in water for 3 h. On the ultra-clean bench, they were rinsed with 75% alcohol for 45 s and cleaned with sterile water for 1 min. Then petioles were sterilized with 3% explants disinfectant (Cygnus) oscillating for 8 min and washed twice with sterile water for 1 min each time. After cleaning, sterile filter paper was used to absorb moisture from the petioles surface. The petioles were cut into 0.50 ± 0.05 cm and inoculated into MS medium containing 1.00 mg·L−1 6-BA + 0.50 mg·L−1 NAA + 30 g·L−1 sucrose + 7 g·L−1 agar + 0.05 g·L−1 activated carbon (pH 5.7−5.8), which medium is hereinafter referred to as M1 medium. They were then cultured under the conditions of 12 h·d−1 light cycle, 2,500−3,000 Lx light intensity, 25 ± 2 °C and 50%−70% relative air humidity. The petioles were induced to differentiate into sterile plants and prepared for use. Robust and consistent tube seedlings were removed and placed on the ultra-clean bench. Leaves were cut into small square pieces about 0.50 cm × 0.50 cm and the petioles were cut into small segments 0.50 ± 0.05 cm long. They were used as callus induction materials.
Effects of different TDZ concentrations on callus induction of leaves and petioles and differentiation of adventitious buds
-
Sterile leaves and petioles were cut and placed on M1 medium with different TDZ concentrations (0.00, 0.50, 1.00, 1.50, 2.00, and 2.50 mg·L−1). Five bottles were set in a treatment and each bottle had six explants. Each treatment was repeated three times. A total of 90 explants were included in this experiment. The six experimental groups were named T1−T6. Calli were observed every 5 d after inoculation. The first induction time and color of the petiole calli were recorded. The status, induction rate, differentiation rate and differentiation coefficient of granular calli were observed and recorded within 60 d.
Types and morphological characteristics of callus
-
In the T1−T6 tests, calli induced by leaves and petioles at different stages (green loose, green granular, green compact, and purple loose) and calli proliferating (white villous, purple loose) were observed and photographed under an NScope 2.0 stereoscopic microscope. The conventional paraffin section method adopted by Lian et al.[17] was used as a reference in this experiment. The calli of different periods were cut, fixed, dehydrated, impregnated with paraffin, embedded, and sliced. They were stained by hematoxylin-eosin staining. The images were then observed under an optical microscope (Nikon, Japan). After the sections were stained with hematoxylin and eosin, the nucleus was blue and the cytoplasm was red. According to morphological and cytological characteristics of callus, the characteristics of embryogenic callus were analyzed and identified.
Callus proliferation
-
The induced calli with consistent growth status were placed on an ultra-clean bench. They were cut into the pieces of 0.5 cm × 0.5 cm × 0.5 cm. Then MS medium with different concentrations of NAA (0.20, 0.40 mg·L−1) and 6-BA (0.50, 1.50, 2.50 mg·L−1) were used for culture. The control group was MS medium without adding any hormone (named CK). The six experimental groups were named D1−D6. Three bottles were set in a treatment and each bottle had ten explants. Each treatment was repeated three times. Biomass gain multiple and callus multiplier multiple were measured after 30 d.
Screening rooting medium
-
The adventitious buds with consistent growth status were placed on MS medium with different concentrations of NAA (0.00, 0.30, 0.50 mg·L−1) and IBA (0.00, 0.10, 0.30 mg·L−1). Ten bottles were set in a treatment and each bottle had six explants. Each treatment was repeated three times. The average bulb quantity, rooting rate, average number of roots, and average root length were counted after 60 d. The measurements were calculated after measuring with a centimeter scale ruler.
Transplanting small bulbs
-
The small bulbs were induced in the rooting medium and removed from the culture bottles with a tweezer. The medium from the bulb surface was washed by running water. 75% wettable carbendazim powder and water were prepared into a solution at 1:1,000 and bulbs were soaked for 3 h. Finally, bulbs were transplanted into the substrate (peat soil : vermiculite = 3:1). Each pot was set to six bulbs. They were watered thoroughly after transplanting and conventional water management was carried out. The germination rate was measured every 10 d. A total of three observations were made.
Data analysis
-
Excel and SPSS 20.0 software were used for the statistical analysis of the data. Duncan's new multiple-range test was used for the difference-significant analysis. Relevant indicators were calculated according to the following formula (1)−(10).
Callusinductionrate=TotalnumberofcallusinductionTotalnumberofcalliineachgroup×100% (1) Differentiationrate=TotalnumberofdifferentiatedcallusineachgroupTotalnumberofpetiolesinoculatedineachgroup×100% (2) Differentiationcoefficient=TotalnumberofdifferentiatedadventitiousbudsofcallusineachgroupTotalnumberofdifferentiatedcalliineachgroup (3) Biomassgainmultiple=TheweightofthecallusmassineachbottleTheweightofcallusineachbottleatinoculation (4) Callusmultipliermultiple=Theweightofthecallusmassineachbottle(Callusweightafteradventitiousbudremoval)Theweightofcallusineachbottleatinoculation (5) Rootingrate=NumberofrootsineachbottleNumberofinoculationsineachbottle×100% (6) Averagenumberofroots=NumberofrootsineachbottleNumberofinoculationsineachbottle (7) Averagerootlength=ThelengthoftherootineachbottleNumberofinoculationsineachbottle (8) Averagebulbquantity=TotalnumberofbulbsinducedineachbottleNumberofadventitiousbudsinoculatedineachbottle (9) Bulbgerminationrate=NumberofbulbsproutsTotalnumberofseededbulbs×100% (10) -
Previous experiments showed that petioles began to induce calli at 10 d in the TDZ treatment group. Calli were induced in all petioles at 12 d. Within 60 d, the calli had five states (Fig. 1a−f): white loose, green loose, purple loose, green granular, and green tight. These five states changed in sequence over time. Firstly, the petioles were induced into white loose calli. Then they turned into green and purple loose calli. Finally, some of them were induced into granular calli (Fig. 1g−h). Compared to no TDZ addition, the callus induction effect in the TDZ-containing medium was better. The entire petioles were transformed into numerous calli. More embryogenic calli were induced in the shortest time. When the concentration of TDZ was 0.50 or 1.00 mg·L−1, the granulated callus induction rate was the highest, at 45.48% and 53.33%, respectively, and there was no significant difference between them (Table 1). The granular calli continued to grow on the original medium and began to differentiate into adventitious buds at 18 d. However, the differentiation rate and differentiation coefficient of the treatment group without TDZ were the highest, at 98.67% and 11.19, respectively.
Figure 1.
Callus cultivated from petiole. (a)−(f) Corresponding to the results of petiole induction callus in the T1−T6 group, respectively; (g), (h) Green granular callus. Scale bar = 1 cm.
Table 1. Effects of different TDZ concentrations on callus induction and differentiation of petiole.
Treatment TDZ concentrations (mg·L−1) Callus status Callus volume Granular callus induction rate (%) Differentiation rate/% Differentiation coefficient T1 0.00 White, loose Only produced at both ends
of the wound15.43 ± 5.05de 98.67 ± 1.44a 11.19 ± 0.45a T2 0.50 Purple, granular, loose Produced at whole petioles 45.48 ± 5.07ab 65.00 ± 5.00b 3.19 ± 0.27b T3 1.00 Purple, granular Produced at whole petioles 53.33 ± 7.64a 58.33 ± 2.89c 3.06 ± 0.35b T4 1.50 Purple, granular Produced at whole petioles 32.87 ± 9.31bc 43.33 ± 0.06e 2.39 ± 0.32c T5 2.00 Purple, granular,
partial white looseProduced at whole petioles 7.78 ± 7.69f 52.22 ± 1.93d 2.40 ± 0.18c T6 2.50 Purple, granular,
partial white looseProduced at whole petioles 27.78 ± 1.92cd 52.22 ± 13.88d 3.39 ± 0.49b The data are mean ± standard deviation. Different letters after the same column of numbers indicate significant differences between different treatments (p < 0.05). Effect of TDZ on calli induced by leaves of O. triangularis 'Purpurea'
-
The callus volume of leaves in the medium supplemented with TDZ was larger than that without TDZ (Fig. 2). Among them, 5% of the leaves induced granular-calli, and more than 95% of the calli were green loose. A large number of calli were generated around the wound throughout the leaves. In the medium supplemented with 0.50−2.50 mg·L−1 TDZ, calli were induced in leaves within 15 d, and differentiation began on 24 d (Table 2). When TDZ concentration exceeded 0.5 mg·L−1, leaf differentiation was inhibited. The differentiation rate of leaf callus was less than 30% and the average differentiation coefficient was less than 5. In the control group, calli began to be induced on 24 d, and the differentiation rate reached 97.38%. The average differentiation coefficient of single leaf was 9.23 after 60 d.
Figure 2.
Calli cultivated from leaves. (a)−(f) Corresponding to the results of leaf induction callus in the T1−T6 group. Scale bar = 1 cm.
Table 2. Effects of different TDZ concentrations on callus induction and differentiation of leaf leaves.
Treatment TDZ concentrations (mg·L−1) Callus volume Initial induction of callus (d) Differentiation rate (%) Differentiation coefficient T1 0.00 Only produced around the wound 24 97.38 ± 2.27a 9.23 ± 0.64a T2 0.50 Produced at whole leaves 15 25.56 ± 7.70b 4.26 ± 0.52bc T3 1.00 Produced at whole leaves 15 11.11 ± 1.93cd 4.33 ± 2.75bc T4 1.50 Produced at whole leaves 15 8.33 ± 2.89d 1.5 ± 0.50c T5 2.00 Produced at whole leaves 15 13.33 ± 7.64cd 5.33 ± 3.21b T6 2.50 Produced at whole leaves 15 29.47 ± 32.21bc 2.67 ± 0.95bc The data are mean ± standard deviation, and different letters after the same column of numbers indicate significant differences between different treatments (p < 0.05). Morphological and histological observation of callus induced by different explants
-
It was found that the callus status of O. triangularis 'Purpurea' varied with time. The leaf organs were inoculated on TDZ-containing medium for callus induction. The petioles formed the white granular calli on the 10th day (leaves on the 16th day) (Fig. 3a) and then became the green tight calli (Fig. 3b). At 20−40 d, calli began to turn into green granular calluses (Fig. 3c). When the calli were cultured on the same medium for more than 60 d, the loose embryogenic calli began to transform into tight embryogenic calli (Fig. 3d), which grew slowly and did not differentiate. Calli were divided into the following four types according to external morphology. Callus I was a white villous calli with a tight texture and was not easily broken when touched by tweezers. Callus II was a green loose calli with loose texture and green granular surface and was easy to damage when touched by tweezers. Callus III was purple and green loose calli with a soft texture and could be damaged when touched by tweezers. Callus IV was a tawny calli with a compact texture and fleshy inside and was easy to brown but not easy to damage when touched by tweezers.
Figure 3.
Induction and histological observation of callus from petioles and leaves. (a), (e) Callus I. (b), (f) Callus II. (c), (g) Callus III. (d), (h) Callus IV, scale bar = 1 cm.
Both cell morphologies are seen in Fig. 3. One type of cell was an embryogenic cell. It had the characteristics of small size, tight and neat arrangement, large oblong nucleus, dense cytoplasm, high karyoplasmic ratio, deep staining, and vigorous cell division. Most of these cells appeared at the edge of the callus and had cytoplasm. The other type was a nonembryonic cell, had the characteristics of large and different volume, irregular arrangement, small round nucleus in the center of the cell, thin cytoplasm, low karyoplasmic ratio, shallow staining, and poor cell differentiation ability. Most of these cells had no nucleus. From the perspective of histology and morphology, with leaves and petioles as explants, the white compact callus (Fig. 3e) initially induced on M1 medium was identified as a non-embryonic callus. The green, and purple loose callus and granular callus induced by TDZ were embryogenic (Fig. 3f−g). On the 90th day, callus cells became larger, and embryogenic cells were less. It grew at a slower rate and became less differentiated, then gradually transformed into a non-embryonic callus (Fig. 3h).
Effects of different 6-BA and NAA treatments on callus proliferation
-
To keep the calli in the embryonic stage as long as possible, the combination of 6-BA and NAA with different concentrations was set up to observe and analyze the proliferation of the callus. At 7 d, the calli had a few white villi growing on the outside. Part of the calluses in groups CK, D1, and D3 started browning and were unchanged in other groups. At 14 d, the calli began to grow significantly and many white calli grew on the surface of most calli. The proliferation rate of callus slowed down at 21 d. There was no significant change in callus proliferation at 21−30 d.
The medium combined with NAA (0.20, 0.40 mg·L−1) and 6-BA (0.50, 1.50, 2.50 mg·L−1) had no significant effect on proliferation and callus multiplier multiple. The change in biomass gain multiple was significant. In the D2 and D6 treatment groups, at 30 d, the biomass gain multiple of callus was the highest, at 4.23 and 4.05 times, respectively (Table 3). In the control group without any hormone, extensive browning occurred at the base of the calli (Fig. 4g). The biomass gains multiple and callus multiplier multiple were the lowest, at 1.86 and 1.93 times, respectively (Table 3).
Table 3. Effects of different 6-BA and NAA concentrations on adventitious bud rooting.
Treatment 6-BA concentration (mg·L−1) NAA concentration (mg·L−1) Biomass gain multiple Callus multiplier multiple Callus texture and color D1 0.50 0.20 3.23 ± 0.14b 3.22 ± 0.18a Purple, loose D2 1.50 0.20 4.23 ± 0.16a 3.47 ± 0.12a White, green, few bud points D3 2.50 0.20 3.37 ± 0.16b 3.29 ± 0.15a White, green, few bud points of granular callus D4 0.50 0.40 3.54 ± 0.67b 3.19 ± 0.10a White D5 1.50 0.40 3.43 ± 0.14b 3.33 ± 0.08a White, dense partial bud points D6 2.50 0.40 4.05 ± 0.37a 3.39 ± 0.29a Green, multiple differentiation numbers CK 0.00 0.00 1.93 ± 0.09c 1.86 ± 0.07b Green, browning on the lower part, low proliferation numbers The data are mean ± standard deviation, and different letters after the same column of numbers indicate significant differences between different treatments (p < 0.05). Figure 4.
Callus proliferation effect. (a)−(g) Correspond to the results of callus-induced callus proliferation in D1−D6 and CK groups for 30 d. Scale bar = 1 cm.
Effects of different concentrations of IBA on adventitious bud growth
-
In this study, the optimal growth conditions of adventitious buds were explored, and the effects of different IBA concentrations on biomass gain multiple and plant status were compared. In the M1 medium, with the increase of IBA concentration, the gain multiple of advent buds showed a trend of first increasing and then decreasing (Table 4). When the concentration of IBA was 0.3 mg·L−1, the advent buds had the best proliferation effect and the biomass gain multiple was 13.52. Secondly, when 0.2 mg·L−1 IBA was added, the biomass gain multiple reached 11.60. When the IBA concentration was 0 mg·L−1, the biomass gain multiple of advective buds was the lowest. Therefore, when 0.3 mg·L−1 IBA was added, the adventitious bud growth of O. triangularis 'Purpurea' was the best.
Table 4. Effects of different IBA concentrations on the induction of adventitious buds.
Treatment Concentrations of IBA
(mg·L−1)Biomass gain multiple Plant status CK 0.00 4.51 ± 0.94e Slender petioles C1 0.10 6.92 ± 0.46c Slender and long petioles green leaves C2 0.20 11.60 ± 0.40b Purple petoiles big leaves C3 0.30 13.52 ± 0.41a Green petioles and leaves granular calli C4 0.40 5.95 ± 0.50cd Short plants C5 0.50 5.88 ± 0.41cd Short plants The data are mean ± standard deviation, and different letters after the same column of numbers indicate significant differences between different treatments (p < 0.05). Effects of different concentrations of IBA and NAA on adventitious buds rooting and bulb induction
-
Without NAA, the overall rooting number was less than when NAA was supplemented, and the growth state of the plants was worse. When the NAA concentration was 0.3 mg·L−1, with the increase in IBA concentration, the average number of roots, average root length, and average height showed an increasing trend. When NAA concentration was 0.5 mg·L−1, there was no significant difference in average height, and the growth state was better. The average number of roots generally increased and then decreased, while the average root length showed a trend of gradual decrease (Table 5). However, when the NAA was not added and whether IBA was added or not, the rooting effect was poor and the plant growth was bad. In the treatment group of 0.3 mg·L−1 NAA and 0.3 mg·L−1 IBA, the rooting effect was the best. The rooting rate was 100%. The average number of roots was 28.16 per plant. The average root length was 1.96 cm and the average height was 6.62 cm.
Table 5. Effects of different concentrations of IBA and NAA on rooting.
Treatment Concentrations
of IBA
(mg·L−1)Concentrations
of NAA
(mg·L−1)Rooting
rate (%)Average number of roots
(bar·plant−1)Average root length (cm) Height (cm) Average bulb quantity
(pcs)Root growth
statusR1 0.00 0.00 91.11 ± 2.48f 7.11 ± 0.08f 1.82 ± 0.15abc 5.71 ± 0.31bcd 0.51 ± 0.32cd Lateral roots on the taproot, large leaves R2 0.10 0.00 92.22 ± 3.06e 5.33 ± 0.49g 1.53 ± 0.41abcde 5.10 ± 0.46d 0.95 ± 0.93bcd Few roots, long taproots R3 0.30 0.00 91.11 ± 2.48f 7.05 ± 0.91f 1.43 ± 0.25bcde 6.13 ± 0.10abc 0.00 ± 0.00d Few roots, thick taproots, no lateral root R4 0.00 0.30 93.33 ± 2.36d 6.33 ± 0.49fg 1.18 ± 0.10de 5.52 ± 0.63cd 0.37 ± 0.19d Thick taproots, no lateral root R5 0.10 0.30 91.11 ± 2.48f 12.89 ± 0.87d 1.63 ± 0.12abcd 6.46 ± 0.16ab 1.99 ± 0.79a Very thick taproots, no lateral root R6 0.30 0.30 100 ± 0.0a 28.16 ± 0.51a 1.96 ± 0.16a 6.62 ± 0.55ab 2.12 ± 0.44a Thick roots, no lateral roots,
numerous rootsR7 0.00 0.50 95.56 ± 3.42b 26.08 ± 0.33b 1.88 ± 0.06ab 7.04 ± 0.30a 2.24 ± 0.30a Thick taproots, no lateral root R8 0.10 0.50 94.44 ± 5.48c 28.25 ± 0.80a 1.40 ± 0.11cde 6.63 ± 0.18ab 1.50 ± 0.84abc Numerous and dense roots, but short, no lateral root R9 0.30 0.50 95.56 ± 6.34b 19.85 ± 0.40c 1.15 ± 0.14e 6.84 ± 0.37a 1.85 ± 0.46ab Thin, short, numerous root The data are mean ± standard deviation, and different letters after the same column of numbers indicate significant differences between different treatments (p < 0.05). In the process of inducing rooting, small bulbs grew at the base of the plant. When NAA was not added and the IBA concentration was 0, 0.1, 0.3 mg·L−1, the bulb induction effect was poor (Fig. 5a−c). When the NAA concentration was 0.3 mg·L−1, the number of small bulbs induced by IBA supplemented medium was significantly higher than that without IBA, and the growth state of bulbs was better (Fig. 5d−f). When the NAA and IBA concentration was 0.5 mg·L−1 and 0.00−0.30 mg·L−1 respectively, there was no significant difference in the number of small bulbs induced, and it had a good induction effect (Fig. 5g−i).
Figure 5.
Rooting results at 30 d. (a)−(i) Correspond to the 30 d rooting results of the R1−R9 groups, respectively. Scale bar = 1 cm.
Sterile bulb transplanting
-
Bulbs were removed from sterile bottles (Fig. 6a). The germination rate was 34.31% at 10 d after transplantation. At 20 d, the germination rate was 57.84% and the bulbs grew into healthy plants at 30 d (Fig. 6b). At 45 d of transplanting, the plants could form flower buds and flowers, and the survival rate was up to 100% (Fig. 6c).
-
The selection of appropriate explants is crucial for inducting embryonic callus. The effect of different explants on callus induction is different[18]. High-quality calli are beneficial to the formation of adventitious buds and the improvement of plant tissue culture efficiency[19]. In the callus induction of O. triangularis 'Purpurea', petioles and leaves are the most common explants. In a few cases, bulbs are used as explants for callus induction. Researchers believed that leaves were the best explants for inducing callus and adventitious bud differentiation[20]. Tang et al.[21] discovered that adventitious buds were induced easily when scales were used as explants of Lilium leucanthum. In the appropriate medium, more calli were induced when induction was carried out with scales. However, compared with L. leucanthum, the callus of O. triangularis 'Purpurea' is difficult to induce. When the basal plate was used as explants to induce callus, the callus formation time was longer and the volume was less. Therefore, bulbs and basal plates are not suitable for callus induction. This study selected leaves and petioles of O. triangularis 'Purpurea' as two different explants. The quality of calli induced by them had significant differences. As explants, petioles had the best effect on callus induction. It was consistent with the research results of Suzana et al.[22].
Exogenous plant growth regulators affect embryogenic callus induction
-
Exogenous plant growth regulators are one of the important factors in inducing embryogenic callus[5]. In the study of callus induction, commonly used auxins are NAA, IBA, and 2,4-D[23,24]. The main cytokinins are 6-BA[25] and KT[26]. Rittirat et al.[27] found that when explants were the petioles of O. triangularis 'Purpurea', adding suitable 2,4-D was conducive to callus induction and the acceleration of adventitious buds growth. Xu et al.[26] found that the combination of 0.5 mg·L−1 KT and a certain concentration of 2,4-D was beneficial to callus induction in Hedera nepalensis var. sinensis. Few scholars have used TDZ to induce the callus of O. triangularis 'Purpurea'. TDZ is a synthetic plant growth regulator. It has a significant effect on callus induction and differentiation[28]. A low concentration of TDZ can promote cell proliferation significantly[29]. The studies of Acer rubrum[14], Sophora davidii[15], and Lens culinaris[30] discovered that TDZ was beneficial to the induction of embryonic callus. In this study, the petioles and leaves of O. triangularis 'Purpurea' were used as explants for induction. In the medium supplemented with NAA and 6-BA, the granular callus induction rate was 15.43%. In the TDZ-supplemented medium, petioles induced white loose callus within 12 d. At 30 d, up to 53.33% of petioles formed close-arranged round granular callus. These results indicated that an appropriate concentration of TDZ could significantly promote the induction rate and callus quality of embryogenic callus of O. triangularis 'Purpurea'. When the petioles were placed on a medium with high TDZ concentration (2.50 mg·L−1) for 30−45 d, the callus induction effect was good and the growth rate was fast in a short time. However, after 60 d of inoculation, calli showed growth retardation and browning. If embryogenic callus status is to be maintained, the TDZ should be evacuated in time. To maintain embryogenic callus status, calli were inoculated on MS medium containing 6-BA and NAA. When the ratio of 6-BA to NAA was 6:1 to 10:1, the callus multiplier rate was the highest, and the multiplication ratio was 3.39 or 3.47 at 30 d. Murthy et al.[31] believed that the growth retardation of callus may result from disrupting the optimal balance of plant hormones in the tissue, or the high concentration of TDZ that increased stress on the plant. Long-term inoculation with a medium containing high auxin would result in somatic embryo deformities and even make calli lose embryogenesis gradually. Therefore, many researchers reduced the concentration of plant growth regulators to a suitable level for the proliferation or maintenance of embryogenic callus status. However, because plant cells at different developmental stages have different sensitivity to hormones, the concentration of growth regulators should be constantly adjusted to maintain embryogenic callus status during the process of proliferation.
Morphology of embryogenic calli in different periods
-
Embryogenic calli grew faster than non-embryogenic calli, and plants were regenerated through the somatic embryogenic pathway[32]. In this experiment, the white villous callus of callus I did not belong to embryonic callus, and the growth rate of callus was slow in this state. This was contrary to the result that the white callus induced in the subculture of Picea mongolica was an embryonic callus[33]. The reason may be that the situation of callus induction was slightly different among different species. Over time, the white callus gradually changed into the loose callus of callus II and the granular callus of callus III. This was consistent with the result that initially induced calli of Triticum aestivum were transformed into embryogenic calli after several subculture times[34].
-
In conclusion, this study established the regeneration system of leaves and petioles of Oxalis triangularis 'Purpurea'. The medium of petiole callus induction was MS + 1.00 mg·L−1 6-BA + 0.50 mg·L−1 NAA + 1.00 mg·L−1 TDZ. Granular calli have a strong ability to divide and regenerate. In this medium, the granular callus induction rate was the highest. The medium for petiole callus differentiation and leaf callus induction and differentiation were MS + 1.00 mg·L−1 6-BA + 0.50 mg·L−1 NAA. Callus proliferation medium was MS + 1.50 mg·L−1 6-BA + 0.20 mg·L−1 NAA. The callus-induced adventitious buds were inoculated onto 1/2 MS + 0.30 mg·L−1 NAA + 0.30 mg·L−1 IBA rooting medium. The rooting rate reached 100% at 30 d. Around 75−90 d, a large number of small bulbs could be induced, and complete plants can be formed. This establishment of an in vitro regeneration system provides useful reference and experimental basis for factory production, genetic engineering and genetic transformation of O. triangularis 'Purpurea'.
This work was supported by 2021 Fujian Agriculture and Forestry University 'Rural Revitalization Service Team' Support Program Project (Grant No. 11899170151), 'Five New Agricultural' Engineering Project released by The Development and Reform Commission of Fujian Province in 2018 [(2018)0438], and Project of Investigation and Regulation of Rare and Endangered Species of National Forestry and Grassland Administration (2020073001).
-
The authors confirm contribution to the paper as follows: study conception and design: Xiao Y, Wu S; data collection: Chen S, Xiao Y, Wang X, Wu K, Shen S; analysis and interpretation of results: Chen S, Xiao Y; draft manuscript preparation: Chen S, Zhai J, Wu S; supervision: Wu S. All authors reviewed the results and approved the final version of the manuscript.
-
All data generated or analyzed during this study are included in this published article.
-
The authors declare that they have no conflict of interest. Shasha Wu is the Editorial Board member of Technology in Horticulture who was blinded from reviewing or making decisions on the manuscript. The article was subject to the journal's standard procedures, with peer-review handled independently of this Editorial Board member and the research groups.
- Copyright: © 2024 by the author(s). Published by Maximum Academic Press, Fayetteville, GA. This article is an open access article distributed under Creative Commons Attribution License (CC BY 4.0), visit https://creativecommons.org/licenses/by/4.0/.
-
About this article
Cite this article
Chen S, Xiao Y, Wang X, Wu K, Shen S, et al. 2024. Establishment of in vitro regeneration system for leaves and petioles of Oxalis triangularis 'Purpurea'. Technology in Horticulture 4: e027 doi: 10.48130/tihort-0024-0026
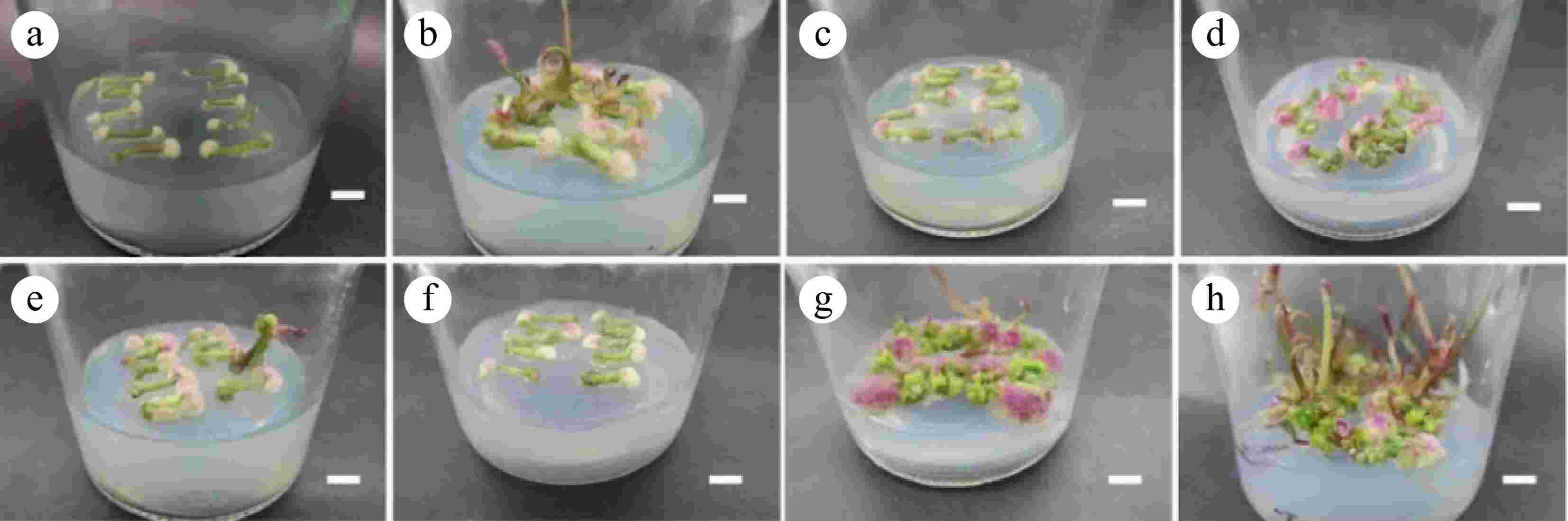