-
Apple (Malus domestica) fruit is widely recognized as one of the most popular pome fruits across the globe, primarily due to its health-promoting compounds such as high dietary fiber, bioactive compounds, flavonoids, and antioxidants[1]. Although, this fruit contains high nutritional and organoleptic properties, its commercial value is limited by loss of quality during postharvest handling and storage. The main causes of apple fruit quality loss and deterioration are high physiological and metabolic activities after harvest that lead to weight loss, color change, softening, loss of acidity, and firmness. In addition, the occurrence of microbial decay remains one of the major causes of apple postharvest loss[2]. Therefore, maintaining the postharvest quality of apple fruit during storage (under retail display cool conditions) and distribution without the use of plastic bags is one of the major challenges for the apple industry[3].
In recent years, edible coatings have been introduced as an environmentally friendly technology with a promising potential to improve the quality and safety of fresh produce. Edible coatings are employed directly on the produce surface that create another layer, thus modifiying the atmosphere around the fruit by providing a semi-permeable barrier to gases and water vapor during handling and storage. This delays the respiration and physiological process that is essential for shelf-life extension. Edible coatings are commonly prepared from eco-friendly and natural biopolymers, such as polysaccharides (starch, pectin, chitosan, cellulose, and alginate) and proteins (zein, casein, whey, and gelatin)[4]. Polysaccharide-based coatings have been shown to have effective film-forming capacity, low cost, and abundant biopolymers[5]. Sodium alginate (SA) is an anionic polysaccharide with polyhydroxy, and carboxyl groups derived from brown algae, a potential biopolymer with unique colloidal properties such as thickening, stabilizing, and film-forming abilities[6]. However, coatings prepared from hydrocolloids such as alginate form strong film, exhibit poor water resistance due to their hydrophilic characteristics, and poor mechanical properties that limit their application in food packaging[7]. Currently, chemical modification of sodium alginate has shown high efficiency by introducing a new fictional group, oxidizing hydroxyl group, or by site-specific modification. This approach is associated with high cost, chemical residues and waste management, hence physical modifications are preferred due to their safety. The potential of cold plasma to modify biopolymers and improve their application has been previously reported[8,9].
Cold plasma (CP) is an emerging technology with wide application in sustainable food systems due to its potential to remove toxic/hazardous chemicals, sterilization, and decontamination efficiency as well as being suitable for heat-sensitive materials[8]. Cold plasma can be generated by exposing gas to an electric field or electromagnetic waves that create a partial gas ionization that leads to the formation of reactive plasma species including atoms, free radicals, electrons, photons, and charged ions. Cold plasma by electrons and ion bombardment effect and free radical production can cause the distruction of DNA. Moreover, CP has been shown as an effective tool to improve the mechanical and functional properties of biopolymers. Thus, is introduced as a promising non-thermal technique to induce physical and chemical changes on the surface of biopolymers than the traditional chemical and physical modification strategies[9]. Consequently, the combined application of cold plasma and edible coating has recently gained attention for its potential to preserve the quality of fresh produce.
Various attempts have been made to determine the combined effects of CP treatment with ECs. For instance, significantly delayed microbial growth was observed on the surface of 'Montery' strawberries coated with sodium alginate and treated with CP store for 6 d at room temperature[10]. Similarly, CP-activated edible coatings were found to limit microbial growth on strawberries, on 'Gala' apples, and 'Gardenblue' blueberries, while maintaining the quality of the produce[11,4]. Meanwhile, the combined effect of chitosan and CP inhibited the loss of firmness and retained the color of fresh pistachio without compromising the aroma and taste[12]. On the other hand, functionalized lily polysaccharide-based edible films with sodium alginate and CP demonstrated a significant improvement of mechanical, barrier, antioxidant, and antimicrobial properties, as well as the thermal stability of the film[13].
Despite the recent advances in CP techniques, previous studies mostly focused on the antimicrobial efficacy of CP-modified edible coatings. Investigations focused on the direct effects of coating and CP treatments on the quality and physiological changes of apple fruit are limited. Therefore, the hypothesis of this study was that: (i) the combined effects of sodium alginate and cold plasma treatments would influence the physicochemical and physiological properties, and microbial population for 'Granny Smith' apples compared to the individual CP treatment, coatings, and control (untreated); (ii) the cold plasma treatment could modify the surface roughness of the apple fruit coated with sodium alginate. To test this hypothesis the following objectives were set: to investigate the integrated effects of low-pressure plasma treatment and sodium alginate coating on the changes in physicochemical and physiological properties, and microbial count as well as microstructural characteristics of 'Granny Smith' apples stored at 15 °C (average retail display condition) for 5 weeks.
-
Sodium alginate powder was purchased from Glentham Life Sciences (Ingoldmells Court, Corsham, UK) and glycerol was purchased from Sigma-Aldrich (St. Louis, MO, USA). Freshly harvested 'Granny Smith' apples were obtained at commercial maturity from a commercial farm, in the Western Cape, South Africa. The maturity index was based on average total soluble solids (TSS) and titratable acidity (TA) of 10.7 ± 0.17 °Brix and 0.9 ± 0.04 g 100 mL–1, respectively. Fruits were transported under cool and well-ventilated conditions to the Agricultural Research Council (ARC) Infruitec-Nietvoorbij, Stellenbosch, South Africa. The apples were carefully sorted to ensure healthy, unblemished, uniform size, and to eliminate decayed fruit. Samples were disinfected with 75% ethanol (v/v) and divided into four categories based on the treatments: (i) Control: as non-coated, and no CP treatment; (ii) CP: treated with CP for 3 min; (iii) CO: coated with sodium alginate, not treated with CP; and (iv) CCP: coated with sodium alginate and treated with CP for 3 min, each was replicated in triplicate. After treatment, all samples were stored at 15 °C for 5 weeks, to simulate the average retail display conditions. Sampling was carried out weekly for all treated and control samples (n = 9).
Preparation of coating formulation
-
The coating solution was prepared according to the method proposed by Belay et al.[10]. Briefly, sodium alginate powder was added to distilled water at 2% (w/v) and the beaker was placed on a magnetic stirrer hot plate (LABOTEC, Model, South Africa) until the sodium alginate had completely dissolved at around 70 °C. Thereafter, 0.25% glycerol (v/v) was added as a plasticizer. The solution obtained was cooled down to ambient temperature, and apples were dipped into the cooled sodium alginate solution for 2 min, removed and drained of excess solution using sterile paper. Thereafter, apples were air-dried at room temperature before either exposure to CP treatment or storage at 15 °C.
Cold plasma treatment and storage
-
Low-pressure cold plasma system (Diener, Zepto (Model 2), Germany) was used to treat the fruit according to the method described by Belay et al.[10]. Plasma was generated inside the vacuum chamber (1.7−2.6 L) with the input of 230 V, 40 kHz, using a feed gas mixture of nitrogen (90%), and oxygen (10%) to generate the plasma. Coated and non-coated apple fruits were subjected to plasma treatment using an output discharge voltage of 80 kV for 3 min. The operational parameters of the cold plasma treatments (output discharge, treatment time, and gas type) as well as the coating application time has been selected based on preliminary investigations. After treatment, fruit samples were immediately stored at 15 °C.
Physicochemical properties
Weight loss
-
Weight loss of apple fruit from the different treatments were measured according to the method by Nsumpi et al.[14]. Fruit weight loss was determined by weighing a fixed set of fruits (n = 9) from each treatment on sampling days using an electronic balance (UWE HGS-15 K, Taiwan) with an accuracy of ± 0.01 g. The difference in the mass between the initial and post-storage measurement was calculated and averaged as a percentage mass loss using Eqn (1):
WL(%)=Wo−WfWo×100 (1) where, WL is the weight loss (%); Wo is the initial weight, and Wf (g) and is the final weight (g) of the apples, respectively.
Total soluble solids, pH, and titratable acidity
-
Apple fruits were juiced using a juice extractor (4294 J700, Braun, China) to determine the total soluble solids (TSS), pH, and titratable acidity (TA) of apple fruit during storage. Apple juice TSS was measured using a pocket-calibrated refractometer (PAL-1, ATAGO, Japan), and the results were expressed as °Brix. Likewise, the TA and pH of the juice samples were obtained from the titration of 60 mL of each fruit juice with 0.333 N of sodium hydroxide (NaOH) at a pH of 8.2, using a Crison Titromatic 1S/2B instrument (Crison Instruments, Barcelona, Spain). The TA results were expressed as g 100 mL−1 of malic acid.
Color
-
Color change on each apple fruit was measured based on the Commission International del' Eclairage (CIE) color system using a digital Chroma-meter (CR 400/410 Konica Minolta Sensing Inc., Japan). Color calibration of the chroma-meter was performed against a white and black tile background before each measurement. Color measurements were taken using individual fruit (n = 3) and data obtained were average of individual color parameters L* denotes the lightness, a* describes red (+)/green (−), and b* for yellow (+)/blue (−). To describe the measured color attributes hue angle (h°), which describes the qualitative attribute of color shades (0° = red-purple and 180° = bluish green), and Chroma (C*), which denotes the quantitative attribute of color intensity were calculated using Eqns (2) & (3).
h∘=(a∗/b∗) (2) C∗=√a∗2+b∗2 (3) Firmness
-
The tissue firmness of individual apples was measured using a texture analyzer (FTA 20, Güss, South Africa). Two opposite sides (left, right) of the fruit were gently peeled, and each was placed on the texture analyzer for a compression test using a probe set with 7.9 mm diameter at a penetration speed of 10 mm·s−1 and a depth of 8.9 mm. All measurements were conducted in triplicate (n = 6) per treatment and firmness was expressed in Newton (N)[2].
Respiration rate (RR)
-
During the 5 weeks of storage, respiration rate (RRCO2) was determined using a closed system method as follows. Apple fruits of known weight were placed into 3 L hermetic glass jars. The jars were developed in-house consisting of three separate jars and lids fitted with silicon septa for outlet and inlet of gas in a closed circuit to avoid reduced gas concentrations inside the jar. About 100 μL headspace gas (CO2) was sampled through the silicon septum on the jar lid using a gas analyzer (Isolcell Oxycarb 6, Italia) after 1 h. The RR was calculated as the amount of CO2 produced per unit mass of the fruit per unit time (mL·kg−1·h−1) using Eqn (4):
RRCO2=(YCO2tf−YCO2tiΔt)Vf/W (4) where,
andYCO2tf are CO2 concentration (%) at time tf (h) and time ti (h), respectively.YCO2ti is RR due to CO2 production in mL·g−1 day Vf is the free volume of the containers and W is the total weight of the product (kg).RRCO2 Microbial analysis
-
Total viable aerobic mesophilic bacteria and yeast and mold were determined using plate count agar (PCA) and potato dextrose agar (PDA) acidified with 10% tartaric acid, respectively. The surface area of the fruit was estimated based on the approach described by Nyamende et al.[15]. Three whole fruits per treatment were placed in separate beakers containing 300 mL physiological solution and placed on a shaker (FMH200Maker, Country) set at 115 rpm for 1 h to adequately remove the microorganisms from the fruit surface. This was followed by the preparation of a dilution series up to 10−3 using 1 mL of diluent transferred into a 9 mL physiological solution. To enumerate the microorganisms, 1 mL of each dilution was plated on PCA and PDA plates in triplicate. The PCA and PDA plates were then incubated at 30 °C for 3 and 5 d, respectively. After incubation, colonies between 30 and 300 were counted on each plate. The results were expressed as log colony forming units (CFU) per surface area of samples (log CFU cm−2). All measurements were carried out in triplicate for each treatment and three levels of dilution (n = 9).
Scanning electron microscopy (SEM) analysis
-
All samples (coated, plasma treated, coated, and plasma treated as well as control) apple fruit surface morphology was evaluated according to the method described by Yanclo et al.[16] with some modification. Small pieces of apple fruit (≈ 3 mm × 3 mm × 2 mm) were taken from fresh fruit with a razor blade, mounted onto a glass slide using carbon-coated double-sided adhesive tape, and then sputter coated with gold (~10 nm thick) using a Leica EM ACE200 Gold Sputter Coater. Samples were loaded and observed with SEM (ZEISS Gemini SEM 300, Carl Zeiss Microscopy GmbH, Oberkochen, Germany) at a voltage of 3.0 kV, 100 IProbe using a magnification of 20 X, 50 X, 100 X, 200 X and 300 X to identify the surface characteristics.
Statistical analysis
-
Two-way analysis of variance was carried out to test the significance of the effects of the coating and plasma treated treatments and storage duration as well as their interactions. All treatments were carried out in triplicate and results were reported as mean and standard deviation. The significant differences (p ≤ 0.05) between the mean values were tested by Duncan's multiple range tests using IBM SPSS Statistics software (version 25.0. Armonk, NY: IBM Corp.).
-
The weight loss of apple fruit increased during storage for all treated and control samples, however, untreated samples exhibited higher weight loss throughout the storage duration (Fig. 1). CP- and CO-treated samples followed the same trend, with no significant difference observed until the fourth week of storage. The percentage weight loss in the CCP-treated samples were lowest throughout the storage duration (p = 0.001). At the end of the storage, weight loss CCP treated fruit was approximately 15.2%, while CO-treated samples was 20.3%, followed by CP treated (23.4%) and control (28.9%) samples (Fig. 1).
Figure 1.
Percentage weight loss of C = control; CO = sodium alginate coating; CP = cold plasma and CCP = plasma-activated coating treated 'Granny Smith' apples during storage at 15 °C for 5 weeks. Mean values (n = 9). Error bars indicate ± standard deviation of means at p ≤ 0.05. Different letters above the error bars indicate the statistically different means at p ≤ 0.05.
The present results agree with a recent study for 'Gardenblue' blueberries and 'Gala' apple fruits in which CP-activated gelatin-carboxymethyl cellulose coating reduced weight loss compared with the control and gelatine carboxymethyl cellulose coated samples without CP treatment[4]. Additionally, the postharvest treatment with radio frequency CP using air at 150 W for 5 s improved the hydrophobicity and water vapor permeability of chitosan/cellulose nanocrystal films, leading to reduced water loss[5]. The reduction in weight loss for CO- and CCP-treated samples in the current study could be mainly due to the thin layer created by the coating on the surface of the fruit, which covered the cracks and pores (as shown by the SEM images), thereby restricting transpiration while permitting gas exchange[3,17]. Fruits are highly susceptible to water loss during storage through the transpiration process that results in weight loss and reduces the fruit quality. Thus, the delayed weight loss observed in the treated samples (coated, plasma-treated, and coated + plasma-treated) highlights the effectiveness of these treatments in minimizing weight loss. Notably, the combination of coating and plasma treatment proved to be the most effective approach. On the contrary to the current study, the study on the postharvest treatment of pomace tomato by using high voltage atmospheric cold plasma (HVACP), 60 kV nitrogen (N2) as a source gas for 15 min resulted in disrupted epidermal structure and led to accelerated weight loss[18]. This could be attributed to the longer exposure of the sample to plasma discharge. It is clear that treatment time plays a significant role, and the plasma operating conditions must be optimized beforehand.
Titratable acidity, total soluble solids, and pH
-
The measured TA declined across all treatments throughout the storage period (Table 1). Comparing the treated and control samples, the highest decline in TA was observed for the control (p < 0.001), from the initial value of ≈ 11.1 g·100 mL−1 to 6.8 ± 0.05 g·100 mL−1 at the end of storage. In contrast, CO-, CP-, and CCP-treated apples retained higher TA values of 7.5 ± 0.23 g·100 mL−1, 8.0 ± 0.15 g·100 mL−1 and 8.2 ± 0.06 g·100 mL−1, respectively at the end of the storage (Table 1). Similarly significant reduction in TA was observed for 'Santa Rosa' plum subjected CP treatment using a DBD system (DBD, 150 kV for 5, 10, and 15 min) and stored 8 °C for 21 d[11]. The decline in TA during storage across all treatments and control is a natural process during fruit maturation and ripening because organic acids act as an intermediate in carbon metabolism and as the key components in response to stress[19]. Higher levels of citrate synthase and NAD-malate dehydrogenase, resulting in the reduced degradation of citric and malic acid of UV-treated 'Xiahui' peach during storage at 1 °C was reported[20]. In another study, the effect of coating (hydroxypropyl methylcellulose, guar gum combined with potassium sorbate) did not show any significant effect on the TA values of orange and tomato during storage at 8 °C[21]. The authors indicated the lack of a clear pattern in the results to conclude the effect of coating on TA.
Table 1. Effects of coating, cold plasma, and cold plasma modified coating on TA, TSS, and pH of 'Granny Smith' apples during storage at 15 °C for 5 weeks.
Quality parameters Storage duration Treatments Control CO CP CCP TA (g·100 mL−1) Baseline 11.13 ± 0.14aA Week-1 10.03 ± 0.04dB 10.44 ± 0.02cA 10.79 ± 0.13bA 11.06 ± 0.03aA Week-2 9.39 ± 0.03fC 9.44 ± 0.01fB 9.56 ± 0.07efB 9.77 ± 0.06eB Week-3 8.78 ± 0.01gD 8.92 ± 0.04gC 9.01 ± 0.04gC 9.24 ± 0.11fgB Week-4 8.40 ± 0.04iE 8.58 ± 0.06hiC 8.65 ± 0.03hD 8.72 ± 0.01ghC Week-5 6.84 ± 0.05kD 7.45 ± 0.23jD 7.96 ±0.15jE 8.24 ± 0.06ijC TSS (°Brix) Baseline 13.11 ± 0.48aB Week-1 13.00 ± 0.62abC 12.70 ± 0.53abB 12.70 ± 0.75abB 12.70 ± 0.60abcB Week-2 13.10 ± 0.10abB 12.97 ± 0.32abB 12.57 ± 0.47abC 12.47 ± 0.38bC Week-3 13.00 ± 0.78aC 12.80 ± 0.95abC 12.50 ± 0.53abC 12.50 ± 0.30bC Week-4 13.73 ± 0.32aA 13.13 ± 0.25aB 12.87 ± 0.31abB 12.77 ± 0.70abB Week-5 13.80 ± 0.10aA 13.70 ± 0.44aA 13.57 ± 0.49aA 13.43 ± 0.25aA pH Baseline 3.32 ± 0.06aA Week-1 3.20 ± 0.03aC 3.15 ± 0.01aC 3.12 ± 0.01bC 3.10 ± 0.01bC Week-2 3.28 ± 0.01aB 3.28 ± 0.01aB 3.27 ± 0.00aB 3.25 ± 0.01aB Week-3 3.31 ± 0.00aA 3.31 ± 0.01aA 3.30 ± 0.01aA 3.29 ± 0.00aA Week-4 3.33 ± 0.00aA 3.33 ± 0.00aA 3.32 ± 0.01aA 3.32 ± 0.01aA Week-5 3.46 ± 0.05aA 3.40 ± 0.02aA 3.36 ± 0.01aA 3.34 ± 0.00aA Data presented means (n = 3) ± standard error, different lower-case letters in rows and upper case in columns represents statistically significant differences based on Duncan's Multiple Range Test at p ≤ 0.05 on TA, TSS, and pH of the fruit. Interaction effects of treatment and storage duration had no significant influence (p > 0.05) on the TSS value was found in all samples (Table 1). Compared to the baseline, no significant difference in TSS were observed for control samples throughout the storage. As demonstrated in Table 1, the TSS declined slightly for CP- and CCP-treated samples from an initial value of 13.11 ± 0.48 °Brix until week 4. A significant reduction of TSS during storage was reported for fresh-cut apple treated with in package DBD CP treatment[22], this could be due to the fact that fresh-cut fruit have higher physiological activities and molecular responses compared to whole fruit. Similarly, the TSS content decreased for strawberries MA packed with nano composite film using 15 kV DBD plasma treatment for 5, 10, and 15 min[11]. The degradation of TSS could be linked to the presence of molecular degradation that can be led by the presence of O2 radicals generated during CP treatment. Contrary, the enhancement of TSS values were observed for 'Santa Rosa' plum fruit after CP treatment (DBD, 100 kV)[11]. However, due to lack of literature regarding the combined or synergetic effects of cold plasma and coating on the quality attribute of 'Granny Smith' apple, the comparison of the results is not fully possible.
The pH of all samples slightly (p > 0.05) increased from the initial value of 3.3 ± 0.06 to 3.5 ± 0.05, 3.4 ± 0.02, 3.4 ± 0.01, and 3.4 ± 00 for Control, CO-, CP- and CCP- treated samples, respectively by week 5. As shown in Table 1, the pH of the apple fruits showed a slight decreasing trend until week 3 of storage, while the pH of CCP-treated samples was lower than that of C, CO-, and CP-treated samples (p > 0.05). This suggests that treatment of samples with coating + CP treatments delayed ripening in apples, which was consistent with calculated RRCO2. This agreed with the study by Rashvand et al.[11] which reported strawberry fruit coated with plasma-activated chitosan nanocomposite was able to maintain and delay the change in pH[11]. The authors attributed this observation to reduced RR and water loss brought about by the improved properties of the coating material. In the present study, the pH of the control and the coated samples were relatively similar and different from those treated with CP- and CCP-treated samples. The highest TA and lower pH observed in the present study could be correlated with the low RR induced by CCP treatments of apple fruit.
Fruit color
-
Postharvest treatment of 'Granny Smith' apples with CO, CP, and CCP had a significant impact (p < 0.001) on L*, C*, and b* color parameters after two weeks of storage at 15 °C (Table 2). The results obtained showed an increasing trend in L* for the control and CO samples (p < 0.001), while CP treatment and CP-activated coating maintained the initial L* of the apples during storage. The highest L* values for the coated apples agrees with the findings of Belay et al.[2], where postharvest coating with zein and zein-nisin composite films maintained high values for L* in 'Granny Smith' apple fruit during storage. According to Ghadermazi et al.[23], the increase in L* value in the film matrix could be due to the light-scattering effects of the matrix compounds. Although the fruit visually remained green in color regardless of the treatment, the control samples were visually brighter.
Table 2. Effect of different treatments on color attributes and firmness of cv. 'Granny Smith' apple coated with sodium alginate treated with cold plasma and stored at 15 °C and 90% ± 2% RH for 5 weeks.
Quality parameters Treatments Storage duration (weeks) Week-0 Week-1 Week-2 Week-3 Week-4 Week-5 L* Control 61.6 ± 0.13a 61.6 ± 0.51f A 64.8 ± 0.35deA 66.9 ± 1.02cdA 69.5 ± 1.20bcA 70.8 ± 1.32abA Coated (CO) 62.5 ± 0.35fA 65.4 ± 0.82dA 66.3 ± 0.48dB 68.0 ± 0.36bcA 72.2 ± 0.17aA CP 62.3 ± 0.44 fA 62.1 ± 1.25fB 61.4 ± 0.71fC 62.7 ± 0.20efB 63.0 ± 1.82defB CCP 63.4 ± 1.31defA 61.9 ± 1.04fBC 61.5 ± 1.41fC 63.2 ± 0.46efB 62.8 ± 0.79fB a* Control −19.5± 0.47a −20.4 ± 0.58aA −17.9 ± 0.19bC −17.0 ± 0.06aB −16.0 ± 0.77aC −16.1 ± 0.33bB Coated (CO) −19.9 ± 0.37aB −18.4 ± 0.13aB −18.0 ± 0.44aA −16.0 ± 0.13aC −14.6 ± 0.09cC CP −20.0 ± 0.09aA −19.3 ± 0.28aA −18.8 ± 0.66aA −19.5 ± 0.04aA −18.7 ± 0.81aA CCP −19.3 ± 0.25aB −19.8 ± 0.56aA −17.4 ± 0.39bB −18.6 ± 0.11aB −19.4 ± 0.71aA b* Control 42.4 ± 0.43f 44.8 ± 0.22defA 45.8 ± 0.62dA 48.3 ± 0.78bcA 48.9 ± 0.28bA 50.7 ± 1.26abA Coated (CO) 45.0 ± 0.61deA 46.1 ± 0.65cdA 47.8 ± 0.74bcA 49.0 ± 0.46bA 51.0 ± 0.27aA CP 43.8 ± 0.60efB 42.7 ± 1.22fB 42.2 ± 0.45fB 43.0 ± 0.14fB 43.5 ± 1.84efB CCP 43.6 ± 0.75fB 43.1 ± 0.15fB 42.8 ± 0.86fB 44.2 ± 0.16eB 44.8 ± 0.21eB h° Control 114.7 ± 0.34a 114.5 ± 1.04aA 111.3 ± 1.38bcB 109.4 ± 1.04cdC 108.1 ± 0.73dC 107.6 ± 1.94cdB Coated (CO) 113.9 ± 1.01aA 111.8 ± 0.09bB 110.7 ± 0.19cC 108.1 ± 2.98cdC 106.0 ± 2.20dB CP 114.6 ± 0.38aA 114.4 ± 0.12aA 114.0 ± 1.31aA 114.4 ± 0.37aA 113.3 ± 1.16abA CCP 112.7 ± 1.06abcA 112.3 ± 0.21bB 112.3 ± 0.21bB 112.7 ± 0.31bB 113.3 ± 0.15abA C* Control 46.7 ± 0.56a 49.3 ± 0.35bcA 49.2 ± 0.55bA 51.2 ± 0.72aA 51.5 ± 0.55aA 53.2 ± 1.07aA Coated (CO) 49.2 ± 0.53bcA 49.7 ± 0.65bA 51.1 ± 0.63aA 51.6 ± 0.48aA 53.1 ± 0.29aA CP 48.1 ± 0.59cdB 46.9 ± 1.00deC 46.2 ± 0.62eB 47.2 ± 0.15dB 47.4 ± 1.40cB CCP 47.9 ± 0.69dC 47.3 ± 0.31dB 47.0 ± 0.64dB 48.0 ± 0.11cB 48.9 ± 0.27bcB Firmness (N) Control 7.00 ± 0.01a 6.82 ± 0.00bD 6.59 ± 0.02cD 6.26 ± 0.02eD 5.19 ± 0.07fgD 4.33 ± 0.15hD Coated (CO) 6.87 ± 0.04bC 6.63 ± 0.01cC 6.32 ± 0.04dC 5.64 ± 0.10fC 4.54 ± 0.36gC CP 6.94 ± 0.01aB 6.69 ± 0.00bB 6.41 ± 0.01dB 5.95 ± 0.06efB 4.67 ± 0.78fB CCP 6.98 ± 0.01aA 6.78 ± 0.03aA 6.48 ± 0.03cdA 6.11 ± 0.08eA 5.16 ± 0.18gA Data presented are means (n = 12) ± standard error, different lower-case letters in rows and upper case in columns represents statistically significant differences based on Duncan's Multiple Range Test at p ≤ 0.05 on peel colour of the fruit. CP = cold plasma treated. A negative a* value was observed throughout the storage irrespective of the treatments. This indicated the green color of the apple. By the end of week 5, the highest a* value (p < 0.001) was observed for coated samples followed by the control, whereas CP- and CCP-treated samples maintained the initial a* value. This demonstrated that these treatments had no detrimental effect on the green color of the apple. This agrees with the study reported for fresh-cut 'Granny Smith' apples where dielectric barrier discharge (DBD) plasma was used for in-package treatment using 30 kV for 1 and 3 min[21]. The authors demonstrated that the cold plasma treatment prevented the incidence of browning after 7 d of storage at 4 °C. A possible reason for the lower values of a* in CP- and CCP-treated apples could be due to the suppressed metabolic activities that led to the inhibition of chlorophyll degradation and carotenoid synthesis[3,24]. Therefore, treatment with CP and CCP could be beneficial for maintaining the green color of 'Granny Smith' apples by regulating chlorophyll metabolism.
A significant increase in C* and b* values resulted in changes in the visual peel color from green to yellowish green for the control and coated apples, suggesting enhanced ripening and chlorophyll degradation. No significant differences were observed in the C* and b* values under CP and CC treated apples in each sampling day, suggesting that the direct CP treatment and activating alginate coating using CP did not alter the color of 'Granny Smith' apples stored at 15 °C for 5 weeks. Similar results were reported for a combined effect of calcium oxide solution washing (CaOW), modified atmosphere packaging (MAP), and cold plasma (DBD, 27 kV, 2 min) treatments used to treat 'satsuma' mandarin[25]. The authors observed the change in color of mandarin fruit (p > 0.05) over time irrespective of the treatments, demonstrating that treatments with CaOW, MAP, and CP influenced the color of the fruit. The color of the apple fruit is one of the main factors that influence consumer acceptability. The present study demonstrated that CP and coating + CP treatments can be effectively used to preserve the color of 'Granny Smith' apples.
Firmness
-
Fruit firmness is an essential quality parameter that is strongly correlated with water loss and metabolic changes within the fruit, including the loss of membrane integrity and degradation of starch and pectin[3]. As presented in Table 2, the firmness of 'Granny Smith' apple for all treated and control samples was reduced during storage (p < 0.001). However, the control sample had the highest reduction of 38%, whereas CP treatment of coated samples resulted in the lowest firmness reduction of 26% by week 5. The results obtained indicated that postharvest treatment with CP and CCP maintained high firmness (p < 0.001) compared to CO throughout the storage duration. These findings suggest that CP treatment alone and in combination with sodium alginate coating was beneficial in preventing loss of firmness. Similar results were exhibited for strawberry fruit coated with CP-activated chitosan nanocomposite film during storage[11]. The authors observed that the CP-activated coating was effective in delaying softening and metabolic reactions of the fruit by forming a semi-permeable barrier that limited gas exchange and delayed water loss.
In the current study, 'Granny Smith' apple fruit treated with CO exhibited lower firmness values throughout the ripening period, compared to CP- and CCP-treated samples, whereas coating treatment was effective compared to the control. The accelerated loss of firmness loss with the postharvest application of CO could be due to the poor water vapor and gas barrier properties of the coating material alone. On the other hand, the effects of CP- and CCP to inhibit the loss of firmness could result from the cold plasma treatment enhancing the surface barrier properties of sodium alginate coating, which influences the physiological properties of the fruit.
Respiration rate
-
The respiration rate of the apples followed a climacteric pattern across all the treatments, with a burst in week 1 (Fig. 2). RRCO2 was highest in the control samples after 1 week of storage followed by CCP, and CO, while CP-treated samples were the lowest. The increased RR in the untreated control samples might be attributed to the ripening process, leading to enhanced O2 consumption by the fruit[3]. Notably, the coated (CO) apples had a moderate RRCO2 response, which suggests that the coating was effective in the modification of the atmosphere around the product[11]. Overall, the RRCO2 of CP- and CCP-treated apples were significantly lower from week 2 until the end of storage (p < 0.001). This observation demonstrates that the postharvest treatments with CP might have contributed to the inhibition of the RRCO2 of the apple fruit. This could be attributed to the improved gas barrier properties induced by the CP modification.
Figure 2.
Respiration rate of C = control; CO = sodium alginate coating; CP = cold plasma, and CCP = plasma-activated coating treated 'Granny Smith' apples during storage at 15 °C for 5 weeks. Mean values (n = 3). Error bars indicate ± SD of means at p ≤ 0.05. Different letters above the error bars indicate the statistically different means at p ≤ 0.05.
In a recent study investigating the effects of CP-activated gelatin-carboxymethyl cellulose (GCMC) on 'Gala' apples and 'Gardenblue' blueberries, it was reported that the treated fruit exhibited a decreased respiration rate[4]. Similarly, the lowest RR observed under CP treatment combined with gelatin-carboxymethyl cellulose coating apple and blueberries compared to the coating and control samples[4]. The authors suggested that CP modification enhanced the mechanical and physical properties of GCMC films such as water vapor, and surface roughness, which affected the respiration and transpiration of the fruits. Furthermore, consistent results were reported for CP-modified chitosan nanocomposite films reducing the RRCO2 of strawberry fruit[11]. The authors suggested that CP treatment improved mechanical and barrier properties of the chitosan nanocomposite films, which led to limited O2 consumption. Thus, the observed respiration inhibitory effect of edible coating combined with CP could be due to the improved surface functionality of sodium alginate by CP treatment that improves the permeability properties, which affects the RR of the fruit. Notably, ethylene production is an important parameter for climacteric produce such as apple fruit. However, this current investigation did not quantify the ethylene production rate for both coated and non-coated fruits. Data on ethylene production of the apple fruits would be suggested for future work to strengthen the discussion on the changes in physiological quality parameters.
Microbial analysis
-
The total aerobic mesophilic bacteria (AMB) count increased significantly (p < 0.001) with storage time for the control samples (Fig 3a). The count increased from an initial value of 3.0 ± 0.13 log CFU cm−2 to 4.2 ± 0.02 log CFU cm−2 by the end of storage at 15 °C (Fig. 3a). The AMB counts were significantly lower for the other treated samples than the control fruit at each sampling time. CP and CCP treatments effectively inhibited the growth of AMB throughout storage resulting in a total count of ≈ 3.2 Log CFU cm−2 and ≈ 3.3 Log CFU cm−2, respectively, at the end of storage (Fig. 3a). These results correspond to the findings on 'Monterey' strawberries coated with sodium alginate[2] and on 'Granny Smith' apples coated with zein and zein/nisin[2,10]. The antimicrobial efficacy of CP and CCP treatments was probably due to the accumulation of reactive species, such as N2+, NO·, and OH·, generated from the plasma treatment[4].
Figure 3.
(a) Total aerobic mesophilic bacteria, and (b) yeast and mold counts of 'Granny Smith' apple, C is control, CO is sodium alginate coating, CP is cold plasma treated, and CCP is sodium lignite coated and cold plasma treated samples storage at 15 °C for 5 weeks. Mean values (n = 9). Error bars indicate ± SE of means at p ≤ 0.05. Different letters above the error bars indicate the statistically different means at p ≤ 0.05.
In contrast, to the AMB response, the yeast and mold count showed an increasing trend throughout the storage period regardless of the treatment. At the end of storage, the highest count was recorded for the control, followed by coated samples, where the counts increased from the initial value of ≈ 2.7 Log CFU cm−2 to ≈ 4.0 Log CFU cm−2, and ≈ 3.9 Log CFU cm−2, respectively (Fig. 3b). Notably, CCP treatments significantly slowed down the growth of yeast and mold on 'Granny Smith' apples (Fig. 3b). The results agree with the study that reported the significant inhibitory effect of CP combined with MAP and CaO compared with untreated samples during 35 d storage of mandarins at 25 °C[24]. Similar results were also reported for fresh-cut 'Whangkeumbae' pears treated with DBD at 60 kV for 5 min using air and stored for 7 d at 10 °C, where the aerobic mesophilic bacteria reduced to below the detection rate[4].
A recent study showed that the postharvest treatment of strawberry fruit with CP-modified chitosan nanocomposite film was more effective in reducing the yeast and mold count of strawberry fruit during postharvest storage[11]. The observed inhibition could be explained by the oxidation of glycoproteins in the cell wall of mold, and the etching of the cell wall surface by CP generated reactive species that can result in cell damage and consequent direct reaction of these species to intercellular biomaterials, such as DNA[24]. Therefore, it can be suggested that CP-modification of ECs has the potential to improve the antimicrobial efficacy of the coating material (sodium alginate), or it could create a non-favorable environment such as CO2 accumulation around the produce. High concentrations of CO2 can be dissolved in the microbial cell, leading to the bursting of cells through explosive expansion[26].
Scanning electron microscopy (SEM) analysis
-
The evaluation of the SEM images of the 'Granny Smith' apple fruit surface demonstrated the presence of different patterns of the amorphous wax layer across the treatments. The morphology of the untreated (control) apple fruit surface displayed an uneven, compact microstructure with small pores with a network of cuticular micro-cracks disrupting the waxy layer (Fig. 4a1). The images obtained using SEM analysis are consistent with previous studies[27,28]. The development of these cuticular micro-cracks could be attributed to the process of fruit growth and maturation[29], due to the pressure buildup in the cuticles, which results in the formation of cracks[27]. These cuticular micro-cracks are characterized as the amorphous wax layer that is disposed on the apple surfaces, which varies in depth, length, and pattern of distribution[27]. The cuticular micro-cracks as artifacts are derived from the rigorous SEM preparation techniques, however, the phenomenon could not be detected with other microscopy approaches. Hence, the authors concluded that the cuticular micro-cracks are naturally occurring with fixed orientation[29]. As the storage progressed at 15 °C, apple surfaces for the control samples changed, the waxy cuticular micro-cracks were closed and became homogeneous and smooth (Fig. 4a2). A similar smooth, continuous and homogeneous amorphous wax layer observation with fewer cracks was reported for 'Jonagold' apples[30], 'Szampion', and 'Jonagold' apples[29]. Based on previous literature, the cuticular waxy layer is produced throughout the life of the fruit and it continues during storage until the byproduct needed is exhausted or tissues die off[31]. At the end of storage week 5, tearing or ripping response of the cuticular waxy layer was observed (Fig. 4a3). It showed the development of wrinkles and minor cracks, indicating rupture of the epidermal layer of the fruit and loss of turgor in the epidermal cells[31]. This phenomenon could be attributed to the fruits enhanced transpiration, and the possible presence of mycelial hyphae in the microcracks and lenticels.
Figure 4.
Scanning electron microscopy (SEM) images of the peel surface of apple fruit at different postharvest handling conditions. (a1−a3) Control (un treated), (b1−b3) plasma treated (CP) fruit peel, (c1−c3) sodium aliginate coated (CO) fruit peel, (d1−d3) sodium alginate coated and plasma treated fruit peel (CCP) at day zero, week 3 and week 5 of storage.
The morphology of the plasma-treated fruit surface (Fig. 4b) noticeably resulted in a closing of cracks compared to the untreated samples. This can be attributed to the specific chemical interaction with the fruit surface, the energy distribution of the applied plasma, or could be as a result of the stress response for the plasma species and uneven plasma etching. The uneven effects of cold plasma treatment on the apple fruit with abrasion and disruption of the surface was clearly visible at day 0 (Fig 4b1). On the other hand, the surface morphology of the coated samples (CO) using sodium alginate exhibited a combination of smooth and rough microstructures with micropores and minor wear (Fig. 4c1) in the first week of storage. At the end of storage, treatment with CO resulted in a brittle microstructure, suggesting the degradation of the coating material (Fig. 4c3). However, it did not alter the visual quality of the fruit (Fig. 5). However, the SEM micrographs showed a consistent increasing degree of smoothness and a high degree of homogeneity on the surface of the apple fruit with the application of cold plasma-activated coating treatments compared to plasma treated and coated samples (Fig. 4d). The coated and plasma-activated fruit surface clearly showed the formation of pinholes on the surface of the sodium alginate coating. Based on the results obtained, fruit samples treated with CP-activated coating (CCP) had a smooth and uniform layer without pores and blemishes. This result indicated a high rate of dispersion on coating material and cold plasma treatment resulting in no cracks or cavity in the coated surface. It could also attest the level to the presence of cross-linking or intermolecular associations that could be a result of the reactive oxygen species imitated during cold plasma treatment. Plasma treatment also results in polished, clean surfaces and increase the surface energy due to its etching effect by physical sputtering or chemical reaction[32].
-
The study compared the combined effects of a sodium alginate (SA) coating and cold plasma (CP) treatment on the biochemical quality, microbial growth, and microstructures of 'Granny Smith' apples during storage at 15 °C for five weeks. The study further compared the effects between coated and plasma treated with those only coated, only plasma treated, and control (untreated). The incorporation of cold plasma with sodium alginate significantly maintained the biochemical quality and extended the shelf life of fresh 'Granny Smith' apples. The results suggested that the CP + SA coating treatment was the most effective in inhibiting weight loss, and maintaining firmness, retaining color, and slowing accelerated consumption of organic acids. The incorporation of cold plasma enhanced the antimicrobial efficacy of the sodium alginate coating, which was evident with lower microbial counts during the five weeks of postharvest storage compared to the control fruits and coated fruit. Cold plasma and coating treatments independently or combined did not affect the TSS, pH, and b* parameters throughout the storage. Moreover, CP treatment of apple fruit coated with sodium alginate coating increased the stability of surface morphology of the fruit. The present study indicated that cold plasma treatment and sodium alginate as an edible coating could be utilized as a potential method to increase the storage ability of 'Granny Smith' apples. Future studies characterizing the physical and chemical properties of plasma-activated sodium alginate coating films and the underlying molecular response (at the proteomic and metabolic level) of 'Granny Smith' apples to CP + coating treatment during storage is needed.
This work is based upon research supported wholly by research grant from the National Research Foundation (NRF) of South Africa (Grant No. 138128) awarded to Dr. Zinash A. Belay.
-
The authors confirm contribution to the paper as follows: study conception and design: Belay ZA, Caleb OJ; data collection: Mathe S; analysis and interpretation of results: Belay ZA, Caleb OJ, Mathe S, Monyela NF, van Breda VM; draft manuscript preparation: Mathe S, Monyela NF, Belay ZA, van Breda VM, Caleb OJ. All authors reviewed the results and approved the final version of the manuscript.
-
The datasets generated during and/or analyzed during the current study are available from the corresponding author on reasonable request.
-
The authors declare that they have no conflict of interest.
- Copyright: © 2025 by the author(s). Published by Maximum Academic Press, Fayetteville, GA. This article is an open access article distributed under Creative Commons Attribution License (CC BY 4.0), visit https://creativecommons.org/licenses/by/4.0/.
-
About this article
Cite this article
Mathe S, Monyela NF, Belay ZA, van Breda VM, Caleb OJ. 2025. Effects of sodium alginate coating and cold plasma treatment on biochemical changes, microbial load, and microstructure of 'Granny Smith' apples during storage. Technology in Horticulture 5: e001 doi: 10.48130/tihort-0024-0029
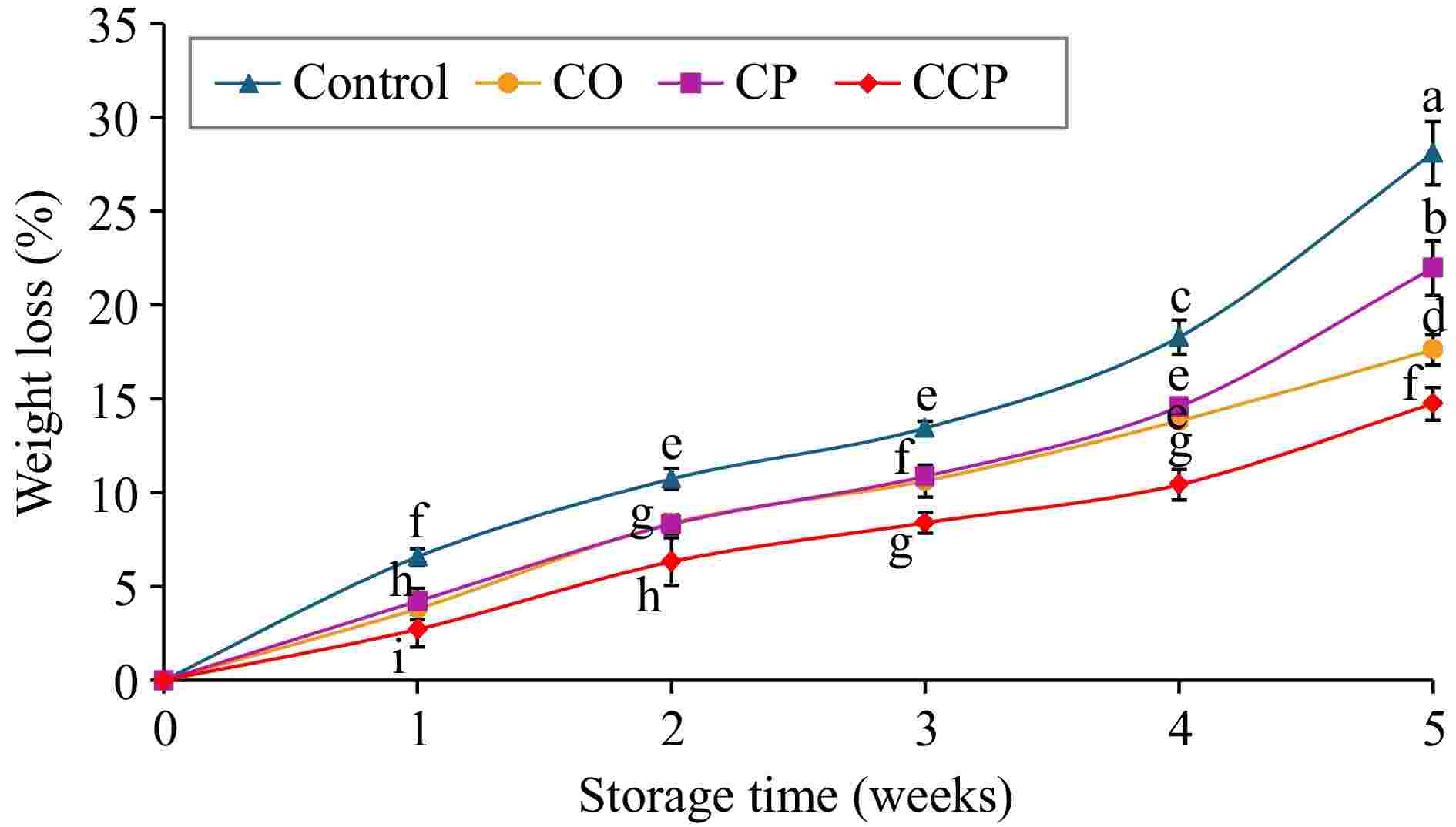