-
Raspberry, belonging to the genus Rubus (known as brambles) in the Rosaceae family, is a fruit species producing small fruits that are usually marketed towards fresh produce, juice, jelly, jams, wine, desserts, tea, and processed or frozen products[1,2]. Raspberry is a woody perennial shrub with biennial canes. The current year cane is called primocane that becomes floricane in the next year. The floricane dies after it produces fruit. Based on the fruiting habit, raspberries can be classified into three types: floricanes that produce the fruit in summer, primocanes that produce the fruit in the fall, and double-cropping ones produce the fruit on both floricanes and primocanes. The raspberry fruit is an aggregate that can be red, black, purple, or yellow/golden with a large variety in fruit size[3]. A USDA (United States Department of Agriculture) survey showed that 6,758 hectares in the United States were dedicated to raspberry production and a total of 81,147 megagrams (89,450 tons) raspberry were produced in 2022 with a market value of 531 million US dollars[4].
Commercial raspberry production in the US is mainly in the Pacific Northwest region including northern California, Washington, and Oregon[5]; however, the demand of locally grown produce/products that are sold at farmer's markets and direct markets has been increasing due to concerns over food quality, nutrition, and food safety[6]. Raspberry is one of the most popular fruit crops for local markets nationwide including North Dakota and other Upper Midwest area. Due to the harsh winters and short growing season in North Dakota, limited raspberry cultivars that are winter hardy with good quality and high yield fruits are available to local growers. The cold climate restricts raspberry production and loss of yield due to cold injury is often observed in the Midwest and Northeast part of the United States[7]. The short growing season in North Dakota also discourages growing primocane raspberry because a large number of berries cannot be harvested before the first fall frost that often occurs in early October (The authors' personal observation). Therefore, the development of cold hardy floricane raspberry or early ripening primocane raspberry with large and flavorful fruit may help increase local raspberry production in North Dakota and other cold regions. Polyploidy plants are those that carry more than two sets of chromosomes that are heritable[8]. Polyploids have been used for plant breeding since 1930s due to the breeding implications and benefits that the polyploidy may have, such as tolerance to pests and diseases, thicker/darker leaves, larger flowers, longer-lasting flowers[8], increased frost tolerance[9], drought tolerance[10], greater chlorophyll content, greater phenolic compound content, greater carotenoid content, increased photosynthesis rate[11], larger fruit size, and different fruit shape[12]. In fruit crop breeding, increasing fruit size and yield as well as improving resistance to biotic and abiotic stresses are often pursued. Thus, the objective of this study was to induce polyploidy of red raspberry cultivars 'Prelude' and 'Polana' using colchicine and oryzalin, two most commonly used anti-mitotic agents for chromosome doubling in plants. 'Prelude' was bred at Cornell University, New York, USA and patented in 2001 mainly for fresh market production[13]. 'Prelude' is one of the earliest-ripening red raspberry cultivars. The fruit on floricanes can be harvested as early as late June in North Dakota. 'Prelude' is a double-cropping cultivar that can also produce fruit on current year growth (primocanes). The fruit on primocanes can be harvested from early September to early November in North Dakota. Canes of 'Prelude' are winter hardy and no severe winter damages were observed in recent ten years in Fargo, North Dakota (USDA zone 4a, −30o F) (unpublished information). 'Polana' is a red raspberry cultivar bred in Poland at the Research Institute of Pomology and Floriculture[14]. 'Polana' is one of the earliest-ripening primocane raspberry cultivars. In North Dakota, the 'Polana' fruit are harvested from late August to late October, making it a good primocane raspberry in the cold region. The ploidy of 'Prelude' and 'Polana' was determined to be diploid (2n = 2x = 14) (unpublished information) using the chromosome counting method[15]. We expect that increase ploidy level of raspberry species may result in improvement of winter hardiness, fruit quality, and yield.
-
Two red raspberry (Rubus idaeus L.) cultivars, 'Prelude' and 'Polana', were used in this research. Tissue cultures of the raspberries were developed in Dr. Dai's lab at North Dakota State University (USA)[16]. Tissue cultures of the two cultivars were maintained by sub-culturing every four weeks in Murashige and Skoog medium (MS)[17] supplemented with 30 g·l−1 sucrose, 5.5 g·l−1 agar (Caisson Labs Inc., Smithfield, UT, 2009), and 3 g·l−1 activated charcoal (Merck KGaA, Darmstadt, Germany). Medium pH was adjusted to 5.7−5.8 before autoclaving at 121 °C and 15 psi for 15 min. All raspberry tissue cultures were maintained in the culture room with a photoperiod of 16/8 h of day/night length, a light intensity of 55 µmol·m−2·s−1 PPF (photosynthetic photon flux), and a day/night temperature of 24/18 °C. Tissue culture conditions in this study were identical unless otherwise noted.
In vitro bud treatment with colchicine and oryzalin for chromosome doubling
-
A total of ten concentrations of colchicine (0, 20, 40, 80, and 160 mM) and oryzalin (0, 80, 160, 320, and 640 µM) solutions were made before the treatment. In brief, 100 ml of liquid MS medium supplemented with 30 g·l−1 sucrose and 1 ml·l−1 PPMTM (Plant Preservative Mixture) (Plant Cell Technology, Inc., Washington, DC, USA) was added to a 300 ml Erlenmeyer flask and autoclaved at 121 °C and 15 psi for 15 min. After cooling, appropriate amounts of 100 mM colchicine (in DMSO) (Sigma-Aldrich, St. Louis, MO, USA) or 100 mM oryzalin came from premixed with a surfactant at 40.4% (Monterey Lawn and Garden, Fresno, CA, USA) were added to the flask to make the final concentration of each solution. Six apical buds (~1 cm) and six nodal buds (~1 cm) were collected from in vitro plants of 'Prelude' or 'Polana' and placed in each of ten flasks. The flasks were covered with aluminum foil, labeled, and placed on an SK-300 Lab Companion shaker (Jeio Tech Inc., Billerica, MA, USA) for a constantly shaking at 110 rpm. After each treatment duration ended (24, 48, and 96 h), two apical buds and two nodal buds were taken from each flask, triple rinsed in autoclaved water and patted dry. Treated buds were then transferred to the 100 ml baby food jar (Sigma-Aldrich, St. Louis, MO, USA) containing 25 ml of MS medium supplemented with 1 ml·l−1 of PPM, 30 g·l−1 sucrose, 5.5 g·l−1 agar, and 10 µM BA for shoot development in the tissue culture room.
In vitro shoots developed from the bud were subjected to ex vitro rooting. Shoots were dipped in a commercial rooting powder Take Root® (United Industries Corporation, St. Louis, MO, USA) for 5 s and inserted into ProMix BX+ (Premier Horticulture Inc., Quakertown, PA, USA) in a 48-hole plug tray covered with a plastic lid. After 4 weeks, plants were being acclimatized by replacing the initial lid (hole-less) with a series of lids with an increasing number of holes on it during a period of four weeks. The rooted and acclimatized plants were subjected to determination of chromosome number.
The experiment of in vitro bud treatment with colchicine and oryzalin was conducted in a randomized complete block design (RCBD) with four replicates in a 2 × 5 × 3 factorial arrangement of two cultivars (with nested tissue apical buds and nodal segments), five concentrations of each agent, and three treatment durations. There were 30 treatments and each treatment had four buds and was repeated four times; therefore, a total of 480 buds were used in this experiment.
Ex vitro root-dipping in colchicine for chromosome doubling
-
Colchicine solutions for root dipping were prepared by adding 100 ml of distilled water into (300 ml) Erlenmeyer flask, then adding appropriate amount of the colchicine stock solution. Plants with roots of 'Prelude' and 'Polana' were pulled out from the ex vitro rooting medium. The lower leaves of the plant were removed and kept only the top 2−3 leaves. The plant roots were trimmed to 5 cm long, gently rinsed with running water for 1 min, and blotted dry. Ten ml of each of four colchicine solutions (0, 0.1, 1.0, and 10.0 mM) was added to a 50 ml disposable plastic tube (Corning Incorporated, Corning, NY, USA). Two plants were inserted into a tube placed in a test tube rack with the root submerged in colchicine solution for a 4 or 8 h colchicine treatment. The tube rack was covered with aluminum foil. There were 16 treatments (2 cultivars × 4 concentrations × 2 durations) and three tubes per treatment (treated as replication). After treatment, plant roots were rinsed three times with distilled water in three 300 ml magenta boxes with 10-min intervals. Rinsed plants were then planted in a seedling tray filled with ProMix BX+ mycorrhizae (Premier Horticulture Inc., Quakertown, PA, USA). The trays were placed in the tissue culture room for plant re-growth.
The ex vitro root dipping experiment was conducted as a RCBD with three replications in a 2 × 4 × 2 factorial arrangement with two cultivars ('Polana' or 'Prelude'), four colchicine concentrations (0, 0.1, 1.0, or 10.0 mM), and two durations (4 and 8 h). There were 16 treatments and each treatment had one plant with three replications; therefore, a total of 48 plants were used in this experiment.
Determination of chromosome number
-
New emerging shoot tips from the plants recovered from the chemical treatment of either in vitro buds or ex vitro root dipping were collected. The meristem of the shoot tip was squashed on a microscope viewing plate covered with a slide (VWR international, Randnor, PA, USA). Acetocarmine (Sigma-Aldrich, St. Louis, MO, USA) solution was used to stain the chromosomes. Chromosome detection was carried out on OLYMPUS CX41 microscope (Leeds Precision Instruments Inc., Minneapolis, MN, USA) at 40× view. After, plants confirmed to be polyploidy were prepared for DAPI (Vectashield®, Burlingame, CA, USA) image capture following the seven-step process. Step 1, slides with a good image of chromosome profile were dipped in liquid nitrogen for 10 s till the nitrogen stopped boiling around the slide. Step 2, put the slide down on a flat surface and pop off the cover slide using a blade on the corner. Step 3, put the frozen slide into 70% ethanol first for 5 min and air dry using clean blotting paper (Fisher Scientific, Pittsburgh, PA, USA) and repeated the previous step with 95% and 100% ethanol solutions (Decon Labratories Inc., King of Prussia, PA, USA). Step 4, after slide dried, load 13 µl of the DAPI antifade mounting solution to where the original center of the slide was, placed fresh cover slide flat on the DAPI droplet, and covered with a blotting paper. Step 5, one min after the DAPI antifade mounting solution spread, pressed gently on the corner of the cover slide to remove excess solution. Step 6, placed the finished slide into a shadow box to protect the DAPI dye from light, and then stored in the fridge until the image capture, and Step 7, took the shadow box with prepared slides into a dark room and captured the image with OLYMPUS BX51 (Olympus America Inc., Center Valley, PA, USA).
Data collection and analysis
-
Data were collected after new bud growth was detected. The percent of initial buds developing new shoots were recorded as the survival rate. The number of plants with double the chromosome number of all survived plants was recorded as the polyploidy induction rate. Data in the in vitro bud treatment were tested for normality assumptions using PROC UNIVARIATE and homogeneity of variances among main factors (cultivar, chemicals, concentration, tissue type) were tested using PROC GLM for Hovtest (i.e. Levene). All data were analyzed by PROC GLIMMIX (SAS version 9.0401M6, SAS Institute, Cary NC, USA) for survival and polyploidy rates that were separated by the LSMEANS for factors and their interactions with Options lines (α = 0.05) for simple effects differences. Since PROC GLIMMIX was used to specify for specific distribution for correct analysis, only Type III tests of fixed effects for each response variables of ANOVA were calculated rather than the sum of squares and their means square.
Results
Effects of cultivar, concentration, and treatment duration on the survival and polyploidy induction of in vitro buds
-
Results of the in vitro bud treatment showed that the effect of cultivar on the survival rate was significant, whereas no significance was found in either concentration or treatment duration of both colchicine and oryzalin. There were no significant interactions between the cultivar and the concentration and treatment duration of colchicine or oryzalin on explant survival rate (Table 1) and polyploidy induction (Table 2).
Table 1. Type III tests of fixed effects of cultivar, concentration, and duration on the explant survival of red raspberry cultivars.
Source of variation Numerator
degrees of
freedomDenominator
degrees
of freedomF Value P > F Colchicine Cultivar 1 201 4.15 0.0430* Concentration 4 201 0.77 0.5443 Duration 2 6 0.71 0.4700 Cultivar × Concentration 4 201 0.47 0.5867 Cultivar × Duration 2 201 0.28 0.2800 Concentration × Duration 8 201 0.68 0.6800 Cultivar × Concentration × Duration 8 201 0.80 0.8000 Oryzalin Cultivar 1 202 7.58 0.0064* Concentration 4 202 0.71 0.5860 Duration 2 6 1.28 0.2807 Cultivar × Concentration 4 202 1.41 0.3146 Cultivar × Duration 2 202 0.46 0.6292 Concentration × Duration 8 202 0.39 0.9267 Cultivar × Concentration × Duration 8 202 0.49 0.8631 * Significant at α < 0.05. Table 2. Type III tests of fixed effects of cultivar, concentration and duration on polyploidy induction of red raspberry cultivars.
Source of variation Numerator
degrees
of freedomDenominator
degrees
of freedomF Value P > F Colchicine Cultivar 1 67 0.32 0.3209 Concentration 4 67 0.41 0.4139 Duration 2 NA NA NA Cultivar × Concentration 4 67 0.41 0.4139 Cultivar × Duration 2 NA NA NA Concentration × Duration 8 NA NA NA Cultivar × Concentration × Duration 8 NA NA NA Oryzalin Cultivar 1 NA NA NA Concentration 4 NA NA NA Duration 2 NA NA NA Cultivar × Concentration 4 NA NA NA Cultivar × Duration 2 NA NA NA Concentration × Duration 8 NA NA NA Cultivar × Concentration × Duration 8 NA NA NA NA: SAS could not compute data analysis due to no polyploidy values being present at different treatment levels. The effect of cultivar on the survival rate in colchicine treatments was significantly different (Table 1). 'Prelude' had a better survival rate (12.95%) than 'Polana' (7.08%) across all colchicine treatments (Table 3). In the oryzalin treatment, the effect of cultivar on the explant survival rate was also significant (Table 1). Unlike the cultivar response to colchicine, 'Polana' (14.17%) has a higher survival rate than 'Prelude' (6.15%) across all oryzalin treatments (Table 3). There were no significant interactions among oryzalin concentration, cultivar, and treatment duration on explant survival rate (Table 1). Due to no polyploidy plants being produced with oryzalin, no probability rate was calculated in SAS ANOVA (Table 2).
Table 3. Effect of cultivar on the explant survival rate in the colchicine and oryzalin treatment.
Cultivar Survival rate (%) Colchicine treatment Oryzalin treatment 'Prelude' 12.95a* 6.15b 'Polana' 7.08b 14.17a * The same letter followed behind indicates no significance at α < 0.05. A tetraploid plant of 'Prelude' was identified from the treatment of 160 mM colchicine for 96 h with a sample size of 16 plants. Chromosome counting indicated that the plant contains 28 chromosomes in the meristem cell (2n = 4x = 28) (Fig. 1).
Figure 1.
DAPI comparison of (a) diploid 'Prelude' (2n = 2x =14) and (b) tetraploid 'Prelude' (2n = 4x = 28). Bar = 10 µm.
Effects of cultivar, colchicine concentration, and treatment duration on plant survival and polyploidy induction in root dipping treatment
-
Results showed that there were no significant differences in plant survival rate among cultivar, colchicine concentration, and treatment duration. No interactions between these factors were detected (Table 4). There was a trend of decreasing survival rate as an increase of colchicine concentration (Table 5). However, significant effects of cultivar (α = 0.1) and colchicine concentration (α = 0.5) on polyploidy induction were detected and an interaction between cultivar and concentration was also significant at α = 0.05 (Table 6).
Table 4. Type III tests of fixed effects of cultivar, concentration, and treatment duration on the plant survival in the root dipping treatment of raspberry cultivars.
Source of variation Numerator
degrees of
freedomDenominator
degrees of
freedomF Value P > F Cultivar 1 32 0 1.0000 Concentration 3 32 1.89 0.1513 Duration 1 32 0.33 0.5677 Cultivar × Concentration 3 32 0.22 0.8802 Cultivar × Duration 1 32 1.33 0.2568 Concentration × Duration 3 32 1.00 0.4055 Cultivar × Concentration × Duration 3 32 0.67 0.5787 Table 5. The effect of colchicine concentration on plant survival rate in root dipping treatment of raspberry.
Colchicine (mM) Plant survival rate (%) 0 100a* 0.1 100a 1.0 97.92a 10 89.58a * The same letter followed behind indicates no significance at α < 0.05. Table 6. Type III tests of fixed effects of cultivar, concentration, and treatment duration on the polyploidy induction in the root dipping treatment of raspberry cultivars.
Source of variation Numerator
degrees of
freedomDenominator
degrees of
freedomF value P > F Cultivar 1 32 4.00 0.0540** Concentration 3 32 4.00 0.0159* Duration 1 32 0 1.0000 Cultivar × Concentration 3 32 4.00 0.0159* Cultivar × Duration 1 32 0 1.0000 Concentration × Duration 3 32 0 1.0000 Cultivar × Concentration × Duration 3 32 0 1.0000 * Significant at α < 0.05. ** Significant at α < 0.1. In the root dipping study, a polyploidy induction rate of 16.67% was achieved in the treatment of 10 mM colchicine for 'Prelude' (Table 7), indicating the different response of cultivars to colchicine. The result also indicates that an increase of colchicine concentration is needed for future polyploidy induction in red raspberry cultivars when ex vitro root dipping method is used.
Table 7. The effect of cultivar × colchicine concentration on the polyploidy rate in root dipping treatment of raspberry cultivars.
Colchicine (mM) Polyploidy induction rate (%) Cultivar ‘Prelude’ Cultivar ‘Polana’ 0 0b* 0b 0.1 0b 0b 1.0 0b 0b 10 16.67a 0b * The same lowercase letter indicates no significance at α < 0.05. -
A polyploidy plant is a plant that carries more than double sets of chromosomes that are heritable[8]. Polyploidy plants possess certain traits, such as tolerance to pests and diseases[8], increased frost tolerance[9], drought tolerance[10], increased photosynthesis rate[11], larger fruit size, and different fruit shape[12], that benefit raspberry breeding and germplasm enhancement. A survey on the field-grown tetraploid red raspberry seedlings showed variations in flower size and fertility, fruit size, seed size, and leaf morphology between diploid and tetraploid raspberries[18]. Such polyploidy benefits could be utilized to potentially increase the plant yield quickly compared to standard breeding procedures[8]. The Rubus species are cytologically complex and their ploidy levels range from 2x to 14x with the basic chromosome number being seven[19−21]. However, in the subgenus Idaeobatus (raspberries), polyploidy is not common and most species are diploid[21] as well as in raspberry cultivars[22]. In this study, a polyploidy induction system was developed through testing two anti-mitotic agents, colchicine and oryzalin and two red raspberry cultivars 'Prelude' and 'Polana'. The ultimate goal of this research was to develop polyploid raspberries with enhanced winter hardiness, good yield, and fruit quality that can be used as new cultivars or unique germplasm for breeding new raspberries for the Upper Midwest and other cold regions of the United States.
In this study, two different ways were adopted for polyploidy induction: in vitro and ex vitro. In the in vitro way, both apical and lateral (nodal) buds were collected from in vitro plants and treated with colchicine or oryzalin under in vitro conditions. Treated buds were then placed back to tissue culture medium for recovery. New shoots grew from the bud were ex vitro rooted and subject to chromosome counting. In the ex vitro way, in vitro shoots were ex vitro rooted and acclimated. Polyploidy induction was conducted by dipping the root system in a colchicine solution under ex vitro conditions. Treated plants were recovered ex vitro and subject to chromosome counting. This study indicates that polyploidy induction in raspberry species can be achieved from both in vitro and ex vitro methods. However, differences in cultivars responding to the anti-mitotic agents and their concentrations were found. One tetraploid plant of 'Prelude' was produced in the in vitro treatment of 160 mM colchicine at 96 h duration that was a combination of the highest concentration and longest treatment time. No polyploid plants were developed from other colchicine treatments and from all treatments of colchicine and oryzalin for 'Polana', indicating the differential responses of raspberry genotypes to anti-mitotic agents and the concentration and treatment time, which agrees with findings in other research that polyploidy induction can be influenced by genotype[23]. Touchell et al.[8] reported that higher concentrations of colchicine and oryzalin lead to a greater polyploidy rate, which was confirmed in this research with colchicine. An increase of the concentration and/or the treatment time may result in a high polyploid induction rate in raspberry species, which should be considered in future research. The result from the root dipping treatment showed a higher polyploidy induction rate (16.67%) than in vitro bud treatment. Two tetraploid plants of 'Prelude' were developed from the treatment of 10 mM colchicine that was the highest colchicine concentration in the ex vitro root dipping treatment, indicating that increasing colchicine concentration is necessary for polyploidy induction using root dipping method. Although there were no significant factors affecting survival rate that might be caused by small sample number and less replicates, a trend where survival rate decreased with an increase of colchicine concentrations in root dipping was observed; therefore, increasing the sample and replicate numbers, optimizing concentration and treatment duration of colchicine (or oryzalin) is also necessary in future endeavor of polyploidy induction of raspberry species.
In this study, all three tetraploid plants of 'Prelude' were induced by colchicine. While colchicine is the most used anti-mitotic agent for ploidy manipulation in plants, oryzalin, an herbicide, is also one of the anti-mitotic agents used for chromosome doubling, particularly in woody or semi-woody plants, including several species in the Rosacea family[8,24] although oryzalin had no success creating polyploidy of 'Polana' and 'Prelude' raspberry in this study. Thus, the applicability of oryzalin to ploidy manipulation of raspberry species needs to be further investigated because oryzalin is more cost efficient and environment friendly than colchicine.
In conclusion, the effects of anti-mitotic agent (colchicine and oryzalin), treatment method, and treatment duration on polyploidy induction of two red raspberry cultivars were determined. A total of three tetraploid plants of 'Prelude' were induced using colchicine and their ploidy was verified using chromosome counting method. These tetraploid plants can potentially be used as new cultivars or breeding materials; therefore, this study can benefit germplasm enhancement as well as genetic understanding of raspberry and other Rubus species.
-
The authors confirm contribution to the paper as follows: study conception and design: Dai W, Hermanson A; data collection: Hermanson A; analysis and interpretation of results: Hermanson A, Dai W; draft manuscript preparation: Hermanson A, Dai W. Both authors reviewed the results and approved the final version of the manuscript.
-
All data generated or analyzed during this study are included in this published article.
-
The authors would like to thank Dr. Jawahar Jyoti for his help with statistical analyses.
-
The authors declare that they have no conflict of interest.
- Copyright: © 2023 by the author(s). Published by Maximum Academic Press, Fayetteville, GA. This article is an open access article distributed under Creative Commons Attribution License (CC BY 4.0), visit https://creativecommons.org/licenses/by/4.0/.
-
About this article
Cite this article
Hermanson AL, Dai W. 2023. Polyploidy induction of raspberry species (Rubus) using colchicine and oryzalin. Technology in Horticulture 3:22 doi: 10.48130/TIH-2023-0022
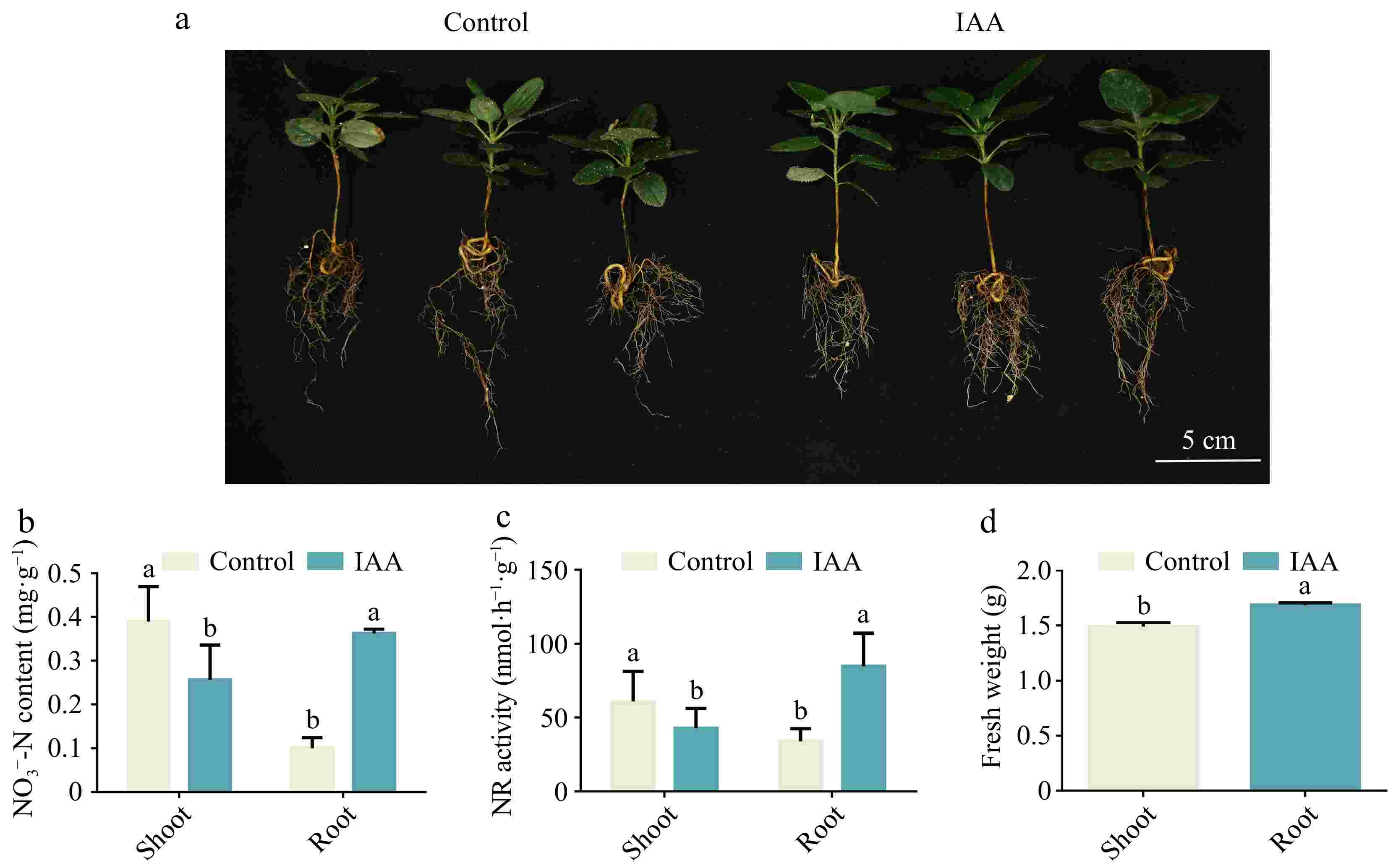