-
In recent decades, commensurate with the growing world population and the mounting threat of climate change, food and nutrition security challenges have, by instinct become much more transparent. The size of arable land areas harvested on a permanent basis decreases because of reasons including urbanization, land erosion, and climate change. This significantly affects the sustainability of agricultural systems[1]. In a similar vein, the decreasing amount of arable land in conjunction with the increasing demand for food has created a dire situation where increasing productivity is the only course of action that takes an integrated and environmentally sustainable approach.
Seed quality is now a key area where farmers take steps to improve their yields and there is also a need to support them in dealing with stress caused by climate change[2]. Both pre- as well as post-harvest environmental conditions affect seed quality. The production of high-quality seeds is an expensive process, more so than the production of high-quality seeded crops. Also, the ideal maintenance of these seeds is a continuous and expensive process. The viability, vigor, and germination ability of quality seeds are reduced under various stressed conditions, leading to poor seedling establishment. These factors are crucial indicators of seed quality, directly impacting yield potential. High germination rates paired with strong seedling vigor are the foundation for quality seeds that ensure robust crop stands and contribute to good yield. Therefore effective strategies to manage and mitigate such damage are crucial to preserve seed quality and ensure optimal germination and vigor[3]. Seed deterioration, initiated by adverse environmental conditions in the field, continues through storage, also impacting seed quality. This deterioration, accelerated by long-term air-dry storage, leads to carryover or marginal seeds with reduced quality.
Consequently, initiatives should be devised that will make it possible to improve the seed quality and enhance the tolerance of plants to biotic and abiotic stresses. One of the promising methods that have emerged recently is using priming techniques, as these produce positive results by improving seed health, germination, and stress tolerance of crops over conventional technology[4]. While multiple primary methods are available in seed quality enhancement techniques, the nanopriming technique stands out as the most advanced and efficient, as opposed to the conventional primary methods. The application of nanomaterials to augment priming responses known as nanopriming, has recently drawn a lot of interest by researchers because of the special qualities and capacity of nanomaterials. Every stage of agriculture, including germination, fertigation, priming, crop protection, seed storage, and post-harvest could benefit from increased agricultural output and quality due to nanotechnology[5]. Higher reproducibility and reliability can result from this enhanced stability in priming applications. While using seed treatment technology, it was found that nanopriming has improved germination rate, seedling vigor, plant tolerance to stress factors, as well as productivity[6].
Two distinct approaches have emerged in the nanopriming technique: chemical-based nanopriming and plant-based nanopriming. To boost priming efficiency, the small size and high surface area to volume ratio (A/V) of nanoparticles can promote improved penetration, cellular absorption, and interaction with the targeted sites[7]. While the chemically-synthesized nanoparticles, in particular, have shown great promise in nanopriming applications. The small size and high surface area-to-volume ratio of nanoparticles can facilitate enhanced penetration, cellular uptake, and interaction with target systems, leading to improved priming efficacy[7]. Chemically synthesized nanoparticles have been thoroughly studied for their potential in nanopriming, and plant-derived nanomaterials and present a strong and environmentally friendly substitute. A cutting-edge agricultural practice called 'green nanopriming' uses nanoparticles which make it an eco-friendly manner by which to improve seed germination and seedling health. Because of its ability to increase crop output sustainably, this strategy is becoming more and more popular. These plant-based nanoparticles often referred to as phyto-nanoparticles, possess inherent biocompatibility, biodegradability, and natural functionalities that can be leveraged for priming applications. While the potential of chemically synthesized nanoparticles in nanopriming has been extensively investigated, plant-derived nanomaterials offer a potent and sustainable alternative.
This review paper provides a comprehensive analysis of the nanopriming technique and its potential to address the challenges faced by modern agriculture. We will explore the distinct characteristics, mechanisms, and applications of these two nanopriming paradigms, highlighting the unique advantages and trade-offs of each approach. Furthermore, we will delve into the environmental impact, biodegradability, and life cycle considerations of the respective nanomaterials, as well as the potential for circular economy and green chemistry principles to be integrated into nanopriming technologies.
-
Seed priming, a method credited to the Greeks has attracted attention from researchers over the years[8]. A study by May et al.,[9] demonstrated that seeds treated and dried under controlled circumstances exhibited germination rates when encountering challenges. The application of solutions containing salts like NaCl, K3PO4 and KNO3 was found to stimulate the growth of tomato seedlings[10]. The term 'priming' was coined by Heydecker et al.[11] in 1973 to describe the practice of soaking and drying seeds before planting. The team further delved into seed priming as a technique to improve germination under conditions involving treating seeds before planting in an environment to trigger germination without root emergence, as noted by Kaur et al.[12] in 2002, and Giri & Schilinger[13] in 2003 (Fig. 1).
-
Priming is a water-based technology that enables controlled rehydration of seeds to initiate the metabolic processes typically activated during the early stages of germination (referred to as 'pre-germinative metabolism'), while preventing the complete transition of the seed into germination. Consequently, the priming treatment must be terminated before the loss of desiccation tolerance[14]. The rehydration process triggers various crucial cellular mechanisms, including the de novo synthesis of nucleic acids and proteins, ATP production, accumulation of sterols and phospholipids, and the activation of DNA repair and antioxidant mechanisms[15,16] (Fig. 2).
-
During the process of priming, different sub cellular changes occur such as biochemical, molecular, and physiological during germination. This is due to the slow imbibition of water by seeds but ceases the water uptake before radicle protrusion. Varier et al.[17] noted that several proteins like globulin, and cruciferin are only detected during priming. Furthermore, the enzymatic activities like catalase, peroxidase, esterase, phosphatase, superoxide dismutase activities, and α- amylase were higher in primed seeds as compared to unprimed seeds[18,19]. On the contrary, the lipid peroxidation activity reduced due to priming. After the imbibition process (Phase-I), DNA repair, cell division, starch break down, and repairing of cellular damage that may occur during maturation, dehydration, and storage[20]. Sathish[21] found no significant difference in DNA content up to 6 h of germination as a result of priming. However, at 12 h of germination, there was a twofold increase in DNA content in maize genotypes that were primed with 1% KH2PO4 for 6 h. Subsequently, the rate of increase in DNA content at 24 and 48 h of germination was lower compared to the rate at 12 h of germination. The pattern of water uptake during germination and priming is similar and the only difference is the rate of water uptake which is slower and controlled to prevent radicle emergence during priming (Fig. 3).
Figure 3.
Schematic representation of seed priming inducing metabolic reprogramming in seeds, leading to enhanced germination and early seedling growth.
Seed priming enhances germination and vigor by triggering various molecular, physiological, and biochemical changes. These include improved reserve mobilization, faster RNA and protein synthesis, increased nutrient content, better membrane integrity, reduced oxidative stress, and enhanced enzyme activity. These factors collectively contribute to the improved performance of primed seeds[22−24].
Gene expression studies in osmoprimed seeds of Brassica oleracea on a cDNA microarray depicted that in primed seeds, genes for serine carboxypeptidase (involved in reserve protein mobilization and transacylation) and cytochrome B (involved in the mitochondrial electron transport) are expressed[17]. Seed priming is also shown to enhance seedling performance by increasing the proportion of cells in the G2 phase relative to the G1 phase. Seed priming resulted in the activation of DNA repair mechanisms, synchronization of the cell cycle in G2, and preparation for cell division[25]. The process of cell division in a germinating seed begins shortly after the radicle (embryonic root) emerges. Seed priming, a technique that extends the duration of Phase II of seed germination, is completed just before the radicle protrudes. Therefore, while seed priming does not directly influence cell division itself, it prepares the seed for the subsequent phase of cell division. Rasool Mir et al.[26] reported the beneficial effect of priming on seedling performance due to the action of replicative DNA synthesis processes before seed germination in hydroprimed maize seeds.
Reactive oxygen species (ROS) also play a crucial role in plant growth and development by acting as signaling molecules. They regulate various processes, including programmed cell death and hormone signaling. ROS, such as superoxide radicals (O2·−), hydrogen peroxide (H2O2), and hydroxyl radicals (OH·), are produced through redox reactions in seeds[27]. Seed priming treatment enhances the ROS mechanism within the seeds, leading to improved seed performance[28].
Priming enhances the activities of cell wall hydrolases as endo-β-mannanase that helps in lowering mechanical constraints during the initial period of germination and radical protrusion[29]. During priming under abiotic stresses, the cellular structures and proteins accumulated during water uptake are known to be protected by specific proteins such as late embryogenesis abundant (LEA) protein and dehydrins[30]. The expression of LEA proteins undergoes sequential changes with a decline during the imbibition phase, upregulation in the dehydration phase followed by degradation during the germination phase[31].
-
Nanopriming, which is a new seed technology, substitutes nanoparticles (NPs) with seed functions and crop productivity. Here the seeds are covered with extremely thin nanoparticles such as metal oxides and polymers and they can use the nanoparticles as delivery tools of beneficial compounds directly to the seed or the emerging seedling. As part of the nanopriming process, nanoparticles quickly permeate the seed coat, increasing the efficiency of the intake of nutrients and water and promoting seed germination[32]. The fundamental material of nanoparticles (organic or inorganic) distinguishes them from one another. The categories of inorganic NPs are further divided as metal (Al, Au, Fe, Cu, In, Mo, Ti, W, Ni, Bi, Co, Si, Ag, Sn, Zn), metal oxide (CeO2, CuO, MgO, SnO2, ZnO, NiO, Cu2O, Al2O3, La2O3, SiO2, In2O3, TiO2, ZrO2) out of which CuO, ZnO, FeO, TiO2, and Ag are often practiced[33,34]. Several genes, particularly those connected to plant stress tolerance, are active during the germination of nano-primed seeds, according to recent publications[6,35,36]. By protecting seeds during storage, enhancing germination, synchronizing germination, and promoting plant growth, nano-priming can also help crops become more resilient to biotic and abiotic stressors, thereby lowering the need for pesticides and fertilizers[37]. Nano-particles have a dual purpose; in addition to being carriers, they are also distributors; they can carry nutrients, growth regulators, or maybe pesticides and allow access to plant cells with these molecules. Such practice may alter the way organic substances are utilized by plants and pests' populations so that fewer resources are being used and a lesser impact on the environment is caused. The nanopriming concept also provides a way in which the efficiency and effectiveness of fertilizers can be enhanced. Nanoparticles can serve as carriers or binders to fertilizers and they will determine when and which nutrients are needed by plants, thus releasing more slowly. This would help to decrease the fertilizer runoff, reduce nutrient losses, and produce higher nutrient use efficiency to bring more sustainable agronomical practices. It offers benefits in the form of selective delivery, enhanced seed performance. and stress tolerance (Fig. 4).
-
The development of electron exchange and improved surface reaction capabilities linked to diverse plant cell and tissue components are the key characteristics of nanoparticles in seed priming. Nano-priming causes the creation of nano-pores in the shoot, aids the intake of water, activates antioxidant and reactive oxygen species (ROS) mechanisms in seeds, and creates hydroxyl radicals, which weaken cell walls and accelerate the hydrolysis of starch[38]. It also triggers the expression of genes called aquaporins, which are involved in the uptake of water and in the mediation of ROS or H2O2 distributed across biological membranes. By stimulating amylase, nanopriming causes starch breakdown and thus accelerates seed germination. The generation of secondary metabolites and stress tolerance are aided by the mild ROS that is induced by nanopriming, which serves as the main signaling cue for a variety of signaling cascade events[6]. In essence, it initiates biological activities intrinsic to the early stages of germination which results in accelerated water absorption, expedited breakdown of starches, softening of cell walls, and weakening of endosperm all leading towards swift development of the embryo along with root-shoot progress. Recently, nanomaterials have been employed as a seed priming agent to enhance plant development and seed germination. They have also been shown to upregulate the defense system by modulating enzymatic activities and preventing stress[39]. Table 1 lists several nanoparticles that have been successfully used in field crops.
Table 1. Effect of various nanoparticles in different field crops.
Sr. no Crops Types of nanoparticles Effect of nanoparticles Ref. 1 Wheat Multi-walled carbon nanotubes Improved seed vigor, plant morphology, and harvest [40] 2 Wheat Iron nanoparticles Improved seed vigor and plant morphology, and increased harvest yield [41] 3 Wheat Silicon nanoparticles Increased biomass and biochemical activity, and reduced cadmium uptake [42] 4 Wheat Zinc oxide nanoparticles Reduced cadmium uptake [36] 5 Wheat Zinc oxide nanoparticles Improved growth biomarkers under salt stress [43] 6 Wheat Copper nanoparticles Abiotic stress resistance development [44] 7 Wheat Silver nanoparticles Increased seed and seedlings vigor [45] 8 Rice Iron (II) sulfide aqua nanoparticles Improved seed vigor and disease resistance [46] 9 Rice Silver nanoparticles Upregulation of aquaporin gene expression, improved seed and seedlings vigor [6] 10 Rice Zinc oxide Improved biofortification [47] 11 Rice Iron nanoparticles Improved enzymatic activity [48] 12 Rice Silver nanoparticles Increased aquaporin gene expression [49] 13 Maize Chitosan nanoparticles containing zinc Improved seed and seedling vigor, and biotic resistance [50] 14 Maize Chitosan nanoparticles containing copper Improved seed and seedling vigor [51] 15 Maize Gold nanoparticles Improved seed and seedling vigor [34] 16 Maize Zinc oxide Increase grain weight, K+ content, and α-amylase activity under salt stress [52] 17 Common bean Zinc nanoparticles Increased biomass [53] 18 Common bean Copper nanoparticles Increased seed vigor, and biomass [44] 19 Common bean Copper nanoparticles High concentrations showed toxic effects on seed germination [44] 20 Soybean Cobalt and molybdenum oxide nanoparticles Improved seed vigor, and plant morphology with increased biomass [54] 21 Soybean Silver nanoparticles Potential antimicrobial activity [55] 22 Tomato Chitosan loaded with gibberellic acid Improved seed vigor and plant morphology with increased biomass [56] 23 Tomato Lignin nanoparticles loaded with
gibberellic acidImproved seed and seedling vigor [57] 24 Tomato Selenium nanoparticles Increased total antioxidant capacity, and chlorophyll content [58] 25 Watermelon Silver nanoparticles Improved seed vigor, and plant morphology [59] 26 Watermelon Iron nanoparticles Increased the activity of plant growth regulator [60] 27 Watermelon Iron oxide nanoparticles Improved plant morphology, reduced phytotoxicity [61] 28 Chili titanium and silver Improved seed vigor, increased disease resistance [62] 29 Chili Zinc oxide nanoparticles High antimicrobial activity [62] 30 Chili Manganese (III) oxide nanoparticles Increased salinity resistance, and antioxidant enzymes [36] 31 Chickpea Lignin nanoparticles loaded with
gibberellic acidImproved seed and seedling vigor [57] 32 Chickpea Zinc oxide nanoparticles Significantly mycelial growth inhibition of Fusarium oxysporum, and increase biochemical activity [63] 33 Chickpea Molybdenum Increased antioxidant enzymes, and harvest [64] 34 Sorghum Iron oxide nanoparticles Increased biochemical activity and biomass, and improved water content in leaves [65] 35 Pearl millet Zinc oxide nanoparticles Antimicrobial resistance [66] 36 Onion Silver nanoparticles Potentially increased bio-chemical activity [67] 37 Onion Gold nanoparticles Improved seed and seedling vigor [68] 38 Pea Platinum nanoparticles Decreased microorganism colonization [69] 39 Spinach Zinc nanoparticles Alleviation of salt stress [70] 40 Rapeseed Cerium oxide nanoparticles Upregulates the expression of salicylic acid biosynthesis under salt stress [71] 41 Cucumber Nanoparticles of water treatment residuals Increase salinity stress tolerance, and biomass [72] 42 Radish Manganese oxide nanoparticles Enhanced nutritional richness [73] 43 Lettuce Zinc, silicon, iron, copper, cerium, and titanium oxide nanoparticles Reducedthe accumulation of reactive oxygen species, and malondialdehyde content under cadmium toxicity [74] -
The fundamental difference between nanopriming and traditional priming is the size and scale of the particles involved. Nanopriming involves particles that are within the nanometer range and for the most part, are less than 100 nm. The nanoscale properties are beneficial and advantageous compared to particles that are in the micrometer scale more commonly in the form of microspheres, micelles, or micro-particles used in traditional priming methods[75]. The reasons are that the nanoparticles are within the size of the target cells and tissues. Consequently, they can efficiently penetrate the target and they also possess enhanced bioavailability and target delivery of the nanoparticles' active agents. Further, the mechanism of action between the two sizes differs vastly. Nanopriming uses the physio-chemical properties of the nanomaterials to achieve target-specific actions and related mechanisms. Nanoparticles' surface characteristics and functions can be engineered to selectively engage with and transport payloads to particular molecular or cellular targets. On the other hand, conventional priming is based on passive distribution and absorption of larger particles, leading to less efficient and less targeted chemical receptivity. Also, improved stability and extended shelf life of nanopriming formulations are a clear advantage over conventional priming techniques. Nanoparticles have small sizes and distinguished surface properties that resist environmental degradation, thus allowing for longer storage and steady performance. Nanopriming, a key application of nanotechnology in agriculture, has shown promising results in enhancing crop productivity, improving nutrient utilization, and mitigating environmental challenges[76−78] (Table 2).
Table 2. Comparative analysis of nanotechnolgy and conventional agriculture technology.
Factors Conventional
technologyNanotechnology
mediated agricultureRef. Yield and quality Low High [67] Crop productivity Low High [79] Nutrient use efficiency Low High [46] Effect on soil health and quality Negative Positive [80] Sustainable crop production Low High [81] Environment remediation Low High [82] Biotic stress and disease tolerance Low High [83] Usage of natural and waste resources for yield attributes Limited Exclusively high [84] salinity stress tolerance Low High [85] Drought stress tolerance Low High [86] Ecofriendly approach No Yes [87] Biomass production Low High [62] Reduction in Mn toxicity Low High [88] Reduction in Cd toxicity Low High [89] -
Natural resources or artificial fabrication are two sources of nanomaterials. Engineered nanomaterials are defined as those that are created artificially or vary from naturally occurring nanoparticles. Engineered nanoparticles (ENs) fall into three broad categories based on their composition: organic nanomaterials, inorganic nanomaterials, and hybrid nanomaterials combining organic and inorganic components. A specific example of a hybrid EN is surface-modified clay, where the surface properties of clay nanoparticles are altered through chemical treatment[90]. Nanopriming based on chemicals is a method in agriculture that utilizes nanoscale materials like nanoparticles of metals or their oxides to precondition seeds before sowing. Abiotic stressors can be detrimental to crops, but they can be effectively mitigated by using metal and metalloid nanoparticles[91]. These minuscule particles can beneficially interact with both the seed and its immediate surroundings, fostering enhancement in plant evolution and vigor across its entire lifecycle. The tiny size and distinctive physicochemical characteristics of nanoparticles render them perfectly suited for their uses. By treating seeds with these particles, enhancements in sprouting, seedling's emergence, as well as augmented growth and productivity of the plant are facilitated[92]. This improvement stems from the nanoparticle's capacity to influence metabolic pathways and signaling within the seed. In the realm of environmental remediation, nanopriming techniques have shown promising results in addressing various forms of pollution. Nanoparticles can be engineered to selectively adsorb and remove contaminants, such as heavy metals, organic pollutants, or radioactive materials, from contaminated soil or water sources[82,89,93]. Nanopriming, a technique that involves the utilization of nanoparticles to augment the catalytic activity of materials, has garnered applications across diverse domains, encompassing chemical synthesis, energy production, and environmental catalysis. Nano-catalysts, in particular, have demonstrated the capability to enhance reaction selectivity, diminish activation energy barriers, and accelerate chemical reactions[94]. These advantages are attributed to the elevated specific surface area and surface energy inherent to nanoscale catalysts, which contribute to their heightened catalytic activity. The controlled synthesis of nanocrystals exhibiting high-energy surfaces, coupled with a comprehensive understanding of the synthesis-structure-performance relationships, constitute pivotal challenges in realizing the widespread industrial and environmental deployment of nano-catalysts[95]. More work is needed to understand the underlying mechanics of seed nanopriming, despite its encouraging results and significant potential for application in agriculture. It has been demonstrated that nanoceria seed priming increases salt tolerance in a variety of plants, such as cotton and rapeseed, via modifying plant signaling pathways related to ROS and ion homeostasis[35,96]. This priming method has been found to improve seedling morphological, physiological, and biochemical traits, as well as root vitality, under salt stress[35]. It also increases the activities of α-amylase, an enzyme involved in starch degradation, and reduces oxidative damage in plant tissues[96]. Furthermore, nanoceria seed priming has been associated with an improved maintenance of the cytosolic potassium/sodium (K+/Na+) ratio, which is crucial for salt tolerance. Several known or proposed mechanisms of seed nanopriming have been identified. Firstly, the application of zinc oxide (ZnO) nanoparticles has been shown to reduce electrolyte leakage and increase the activities of superoxide dismutase (SOD) and peroxidase (POD) enzymes[97]. These effects contribute to enhanced oxidative stress tolerance and membrane integrity. Secondly, iron oxide (Fe2O3) nanoparticles have been associated with decreased lipid peroxidation, increased plant-relative water content, and improved photosynthetic performance[65]. These mechanisms aid in maintaining cellular homeostasis and enhancing the plant's overall physiological state. Additionally, the use of silver nanoparticles has been linked to lower percentages of micronuclei and chromosomal abnormalities[98], suggesting a potential role in mitigating genotoxic effects and promoting genomic stability. Moreover, silver nanoparticles have been found to increase α-amylase activity, leading to higher soluble sugar, which supports seedling growth and upregulates aquaporin genes, potentially facilitating greater water uptake[6]. These processes contribute to improved seedling establishment and water management. Furthermore, iron oxide (Fe2O3) nanoparticles have been implicated in reducing the level of 12-oxophytodienoic acid, which aids in breaking seed dormancy[61]. This mechanism promotes germination and early growth stages. These proposed mechanisms highlight the multifaceted effects of nanoparticles in seed priming, encompassing physiological, biochemical and molecular processes that collectively enhance plant performance under various stress conditions.
-
These synthetic nanoparticles have beneficial impacts on the agriculture sector as explained above, but the negative impact has also been observed by these nanoparticles on crop production higher than specific concentrations. Plants that are exposed to nanoparticles (NPs) experience cytotoxic, genotoxic, and phytotoxic abnormalities that result in slowed germination, reduced plant growth, and elongated roots[99,100]. In addition to making plants toxic, they also have an impact on aquatic life, humans, and soil bacteria through the food chain. It was discovered that in the root tips of onions (Allium cepa), silver nanoparticles (Ag NPs) and silver ions (Ag+) reduced the mitotic index and severely chromosomal aberrations[101]. Similarly, exposure to ZnO and CuO nanoparticles caused significant morphological and molecular modifications[102]. Lv et al.,[103] found phytotoxicity due to ZnO nanoparticles size of 9 mm in maize roots, alteration in root tip morphology, cortical collapse and vacuolation, and destruction of the epidermis and root cap of ryegrass. Zn, Cu, Ce oxide nanoparticles leads to chronic toxicity due to dietary intake of metal components in carrot[104]. Asgari-Targhi et al.[105], and Maity & Pramanick,[106] observed inhibited plant growth, seed germination, and gene expression due to chitosan-based polymeric nanoparticles. Through the inhibition of lignin-specific gene expression, Yttrium NPs caused oxidative stress, reduced bud elongation, root elongation, root activity, protein, and phototoxicity[107]. According to Mosquera et al. and Kibbey & Strevett[108−109], the introduction of nanoparticles in soil and plants may have a direct or indirect impact on the diversity and functions of microorganisms. Sułowicz et al.[110] reported that the ZnO and SiO2-based nano-fungicides changed many microbial properties, which in turn affected soil microorganisms.
-
The concept of 'green nanopriming' was introduced as a method to enhance seed germination using environmentally friendly synthesized nanoparticles. This approach emerged as a response to the need for non-toxic alternatives to traditional chemical methods in agriculture[111]. Using nanoparticles (NPs) produced via green chemistry—which uses plant extracts as reducing agents rather than hazardous chemicals—is known as 'green nanopriming'. As an eco-friendly and economical alternative to conventional chemical synthesis, this approach shows promise[111]. For example, extract from kaffir lime leaves was used to create silver nanoparticles (AgNPs), and extract from coriander herbs was used to create copper oxide (CuO) nanoparticles[6,112]. It is then utilized to prime seedlings with these green-synthesized nanoparticles, increasing their germination, and growth potential. The process of creating nanoparticles (NPs) usually involves chemical reduction. However the majority of the lowering agents are extremely hazardous substances. Because plant alternatives are more environmentally friendly than such hazardous reductants, green synthesis of nanoparticles (NPs) has emerged as an emergent technique[113,114]. Green nanopriming works well because it can improve several physiological and biochemical functions in seeds. The creation of nano-pores by nanoparticles can improve the generation of reactive oxygen species (ROS), enable water uptake and increase the activity of hydrolytic enzymes such as α-amylase, which is essential for starch metabolism[6,84]. When taken as a whole, these procedures increase seed germination rates and seedling health. For instance, it has been demonstrated that priming maize seeds with gold nanoparticles (GNPs) greatly raises the emergence percentage and seedling vigour index[6]. The low cost and environmental friendliness of the green-synthesized approach, in comparison to the conventional synthetic method's high energy-intensive, multi-step processes or formulations of harmful chemicals, have drawn increasing attention[115,116]. It synthesizes NPs using plant extracts and among its benefits are low cost, environmental friendliness, and biocompatibility. The plant extracts are obtained by boiling various plant components (leaves, stems, roots, etc.) in distilled water after they have been cleaned with distilled water[67,117]. In a few environmentally friendly minutes, the extracts are simply combined with the metallic salt solution at a particular temperature to transform metal ions into NPs. Green nanopriming is the process of seed priming using green synthetic nanoparticles[117].
Rhamnus triquetra and Lantana camara are used to produce green ZnO NPs, which have antibacterial qualities against microorganisms. Using leaf extract from Piper colubrinum, Santhoshkumar et al.[118] produced biocompatible silver nanoparticles and observed increased seed germination, seedling growth and chlorophyll content in rice. Similar findings were observed by Datta Gupta & Pattanayak in potato, and Acharya et al., in watermelons[59,119]. After encasing curcumin as a priming agent, chitosan nanoparticles effectively reduced the amount of ionic toxicity in wheat seedlings under salt stress by upregulating the machinery of antioxidants (CAT, POD, APX, and SOD), photosynthetic pigments (Chlorophyll (Chl) a, Chl b, total Chl, and lycopene), tannins, flavonoids, and protein content. Zinc oxide nanoparticles synthesized from Citrus limmeta peel extract against plant pathogenic bacteria showed complete elimination of soft and brown rot infections in comparison to non-primed potato tuber slices[120]. Magnesium sulphide nanoparticles (MgS NPs) using an extract from Hordeumvulgare leaves enhanced average root and shoot lengths, as well as accelerated germination in Brassica nigra and Trigonellafoenum graecum successfully[121]. Green nanopriming is successfully effective against insect pest attacks and can be used as nanopesticides. Ibrahim et al.,[122] assessed the entomotoxic properties of silica nanoparticles using rice straw against C. maculatus. Similarly, insecticidal activity was illustrated by Salem[123] using silica nanoparticle with rice husk against Callosobrochus maculatus, Rhythopertha dominica, and Tribolium confusum. The seed quality parameters like germination, seedling fresh weight, shoot length, root length, and vigor indices were enhanced using iron oxide nanoparticle formation with an aqueous plant extract of Salvinia molesta in tomato[124].
-
Despite impressive advancements, it is acknowledged that scientists still lack a thorough grasp of how these nanomaterials may impact the macro- and micro-environments of seeds. Given the current and anticipated levels of exposure, it is concerning that fundamental knowledge about the potential health and safety impacts of manmade nanomaterials on both human and non-human receptors is still lacking. There is no universal rule governing seed nanopriming and there is no discernible pattern in priming responses based on a species' taxonomic position. Certain nanopriming procedures may cause the medium to become contaminated with bacteria and fungi, which could seriously impair the germination of succeeding seeds. Mature seeds take longer to dehydrate than nano-primed seeds, yet a nano-primed seed dries back to its initial moisture content. Numerous scientists have postulated that harsh desiccation techniques modify the results of nanopriming[125]. Nano-primed seed material may therefore be less stable, which results in increased maintenance expenses for farmers and seed firms. Repeated nanopriming treatments can partially reduce seed viability losses in certain situations, but in others, the losses are irreversible. Because germination potential might not be entirely recovered, the need for a second treatment could be both an additional expense and a source of unpredictability[126].
-
Green nanopriming represents a groundbreaking approach to sustainable agriculture, offering a novel alternative to conventional synthetic nanoparticles. This innovative technique harnesses the natural reducing capacities of plant extracts to create eco-friendly nanoparticles, aligning seamlessly with green chemistry principles. Its applications extend beyond crop enhancement to include green nanopesticides, nutrient solubilization, and crop quality optimization. The future of green nanopriming hinges on ongoing research to elucidate its mechanisms and assess potential risks, necessitating interdisciplinary collaboration among experts in nanotechnology, plant biology, and environmental sciences. As this technology matures, it promises to revolutionize agricultural practices, striking a crucial balance between productivity, food security, and ecological preservation. The advent of green nanopriming marks a significant paradigm shift towards an environmentally conscious farming future, potentially transforming how we approach sustainable agriculture on a global scale.
-
The authors confirm contribution to the paper as follows: study conception: Aggarwal S, Mor VS, Malik A; preparation of the first draft: Aggarwal S, Paul D, Tanwar H; preparation of tables and figures: Aggarwal S, Paul D; editing and proof reading of the manuscript: Aggarwal S, Paul D, Tanwar H, Mor VS, Malik A. All authors reviewed the results and approved the final version of the manuscript.
-
All data generated or analyzed during this study are included in this published article.
-
The authors duly acknowledge the support received from the Department of Seed Science and Technology, CCS-Haryana Agricultural University, Hisar, Harynana, India.
-
The authors declare that they have no conflict of interest.
- Copyright: © 2025 by the author(s). Published by Maximum Academic Press on behalf of Hainan Yazhou Bay Seed Laboratory. This article is an open access article distributed under Creative Commons Attribution License (CC BY 4.0), visit https://creativecommons.org/licenses/by/4.0/.
-
About this article
Cite this article
Aggarwal S, Mor VS, Paul D, Tanwar H, Malik A. 2025. Green nanopriming: a comparative analysis of chemical and bioinspired approaches for sustainable agriculture. Seed Biology 4: e001 doi: 10.48130/seedbio-0025-0001
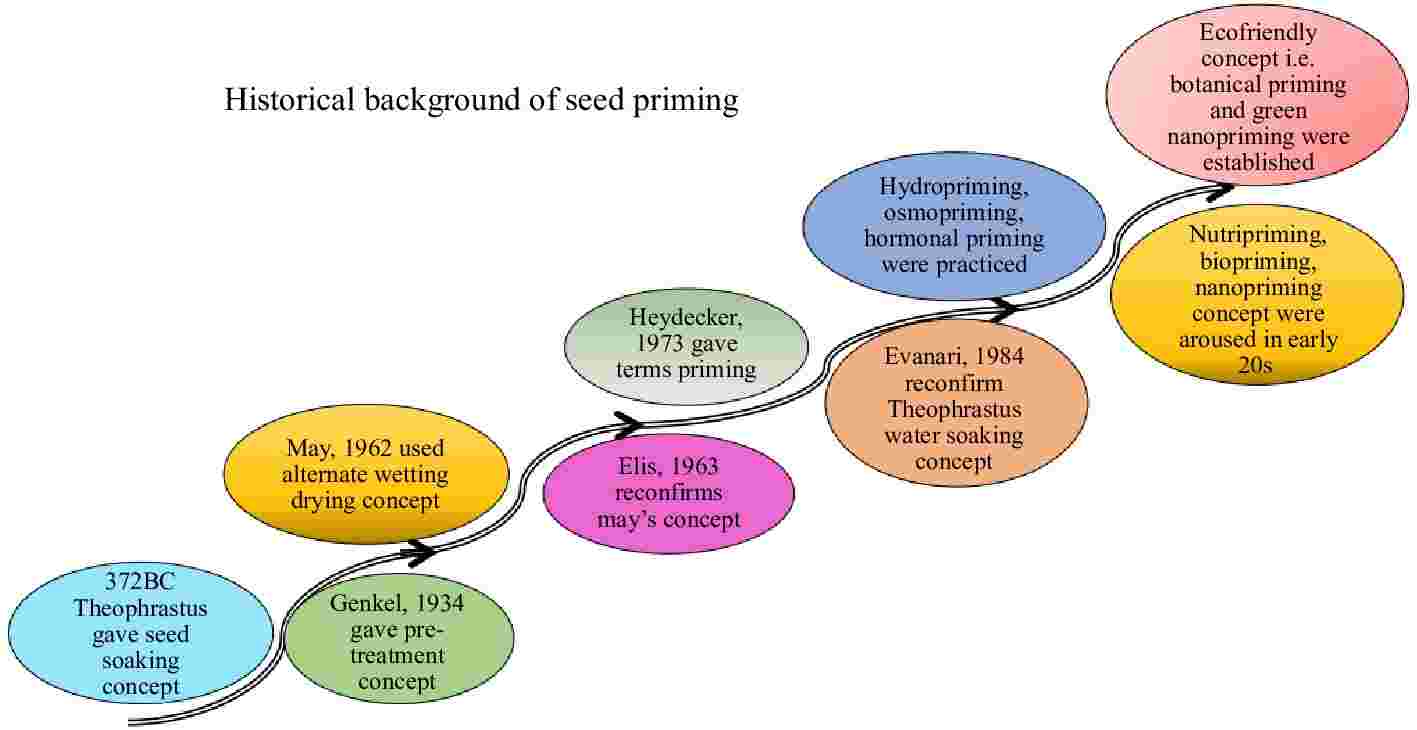