-
Environmental pollutants such as heavy metals, microplastics, and antibiotics have raised significant concerns about agricultural sustainability, ecosystem stability, and human health[1] . Cadmium (Cd) contamination has attracted the most attention because of its high toxicity, widespread pollution area, and easy uptake by plants[2]. Cd enrichment in soils can change soil physicochemical properties, and reduce soil fertility[3]. As a nonessential element for organisms, Cd can influence the normal physiological function of most plants at low concentrations, leading to growth inhibition, metabolic dysregulation, and even plant death[4]. Moreover, Cd can easily be absorbed by crops and vegetables to enter the food chain, ultimately endangering human health[5,6].
To ensure agricultural food safety, remediation of heavy metal-polluted soil is necessary. To date, separate or combined biological, physical, and chemical methods have been developed to remediate contaminated soils[7]. Physical‒chemical techniques are commonly applied in soil remediation because they have quick effects[8]. However, most physical‒chemical techniques are expensive, easily introduce secondary pollution, or destroy soil properties; thus, they are difficult to apply widely and sustainably[9]. In contrast, biological remediation, which decreases heavy metal concentrations or bioavailability in soils through the metabolic activities of organisms[10], has the advantages of low cost, environmental friendliness, and easy management, thereby attracting much interest[11].
Phytoremediation, especially the phytoextraction technique, has become one of the highly anticipated bioremediation methods in recent years because of its simple, environmentally friendly, and cost-effective feasibility[12]. Phytoextraction is dependent on the abilities of plants, especially those hyperaccumulators, to absorb heavy metals and remove them[13,14]. To date, hundreds of heavy metal hyperaccumulators have been discovered worldwide[15] and new hyperaccumulators are being constantly reported[16,17]. However, because heavy metal contents accumulated by plants are determined by both the heavy metal concentrations in plants and plant biomasses, quite a few heavy metal hyperaccumulators with slow growth rates, and low biomasses[18] cannot be practically used for phytoremediation. Additionally, the shape of plants can determine the optimum planting density of plants in the field, thus affecting the removal efficiency of heavy metals, which is also worth paying attention to when screening or breeding plant resources for phytoextraction. To date, laboratory research has hardly simultaneously focused on these factors. In this study, Cd tolerance and accumulation capacities of two Crassulaceae species, Phedimus aizoon and Kalanchoe blossfeldiana were identified. On this basis, a new index, theoretical phytoextraction rate (TPR), integrating plant biomass, heavy metal accumulation capacity, and plant shape and size are proposed to perform a more referential evaluation of their application potential for Cd phytoextraction. This study is expected to provide new plant resources and theoretical methods for heavy metal phytoextraction.
-
Uniform seedlings that were approximately 10 cm high of both P. aizoon and K. blossfeldiana were purchased from a horticultural company located in Yunnan Province, China. The seedlings were neatly transplanted into uniform flowerpots according to the method of Yang et al.[19]. The Cd-free soils and contaminated soils were obtained according to the methods of Li et al.[20], with three replicates of each group. The flower pots were placed in a greenhouse with appropriate watering (500 ml each time, about once every 2 d, tap water without Cd) to avoid soil surface dryness.
Measurement of plant growth
-
After 3 months of growth, the plant size was measured before the plants were harvested. The diameter of the maximum amplitude and the diameter in the vertical direction were used to represent the plant size. After the roots were washed three times with deionized water, the root length was measured. The samples of leaves, stems, and roots were treated as described previously by Li et al.[21].
Chlorophyll content measurement
-
Fresh leaves (top first to third) were harvested to measure the chlorophyll content. Fresh leaves (0.1 g) were soaked in 10 ml 80% acetone for 24 h under darkness. After centrifugation at 12,000 ×g for 10 min, the absorbance of the resulting supernatant was precisely measured at wavelengths of 645 and 663 nm via a spectrophotometer (Youke, Shanghai, China). The concentrations of chlorophyll a (Chla) and chlorophyll b (Chlb) were calculated via formulas described in a previous report by Liao et al.[22].
Detection of reactive oxygen species (ROS)
-
The concentrations of superoxide anions (O2−) and hydrogen peroxide (H2O2) were detected using their corresponding kits (Solarbio, Beijing, China) according to the instructions.
Antioxidant enzyme activity assay
-
The activities of superoxide dismutase (SOD) and peroxidase (CAT) were detected using their corresponding kits (Solarbio, Beijing, China) according to the instructions.
Cd concentration detection
-
The Cd concentrations in dry samples were measured via a previously described method by Li et al.[23].
Index calculation
-
Bioconcentrationfactor(BCF)=SampleCdconcentrationSoilCdconcentration Translocationfactor(TF)=LeaforstemCdconcentrationRootCdconcentration TPR=Cdcontentinabovegroundofindividualplant×OptimumplantingdensitySoilconcentration×(Rootlength×Unitarea×Soildensity)×100% Optimumplantingdensity=UnitareaPlantsize=UnitareaDiameterofmax.amplitute×Diameterinverticaldirection Statistical analysis
-
Bar charts were drawn using SigmaPlot 10.0 software (Systat, San Jose, CA, USA). Significant difference was analysed by SPSS 18.0 software (IBM, New York, NY, USA).
-
After 3 months of growth, the dry biomasses of individual plants of both P. aizoon and K. blossfeldiana were similar between the control and Cd-treatment conditions (Fig. 1a). Similarly, the plant sizes (Fig. 1b) and root lengths (Fig. 1c) of both P. aizoon and K. blossfeldiana were not markedly affected under the Cd treatment compared with those under the control conditions. Consistent with the plant morphological results, it was also found that the concentrations of both Chla and Chlb were not affected by Cd treatment (Fig. 1d).
Figure 1.
Plant growth and chlorophyll content of P. aizoon and K. blossfeldiana under control and Cd treatment (50 mg·kg−1) conditions. (a) Dry biomasses of roots, stems, and leaves. (b) Plant size. The data represent the estimated optimum planting density for each species. (c) Root length. (d) Chlorophyll a (Chla) and chlorophyll b (Chlb) contents. Data represent means ± standard deviations (n = 3).
To estimate the effects of plant morphology and growth rate on phytoextraction efficiency, the biomass, plant shape, and size of individual plant were compared between P. aizoon and K. blossfeldiana. Although the total biomass of individual plant and root length were similar between P. aizoon and K. blossfeldiana during similar growth periods (Fig. 1a, c), the proportions of their aboveground and underground parts were different (Fig. 1a). In addition, the plant size of P. aizoon was much larger than that of K. blossfeldiana (Fig. 1b), since P. aizoon and K. blossfeldiana had scattered and upright shapes, respectively. Based of plant size, it was estimated that the optimum planting densities of P. aizoon and K. blossfeldiana for 3-month growth were approximately 7–8 and 16–18 plants per square meter, respectively (Fig. 1b).
Changes in ROS and antioxidant enzyme activities
-
To further understand the response of P. aizoon and K. blossfeldiana to Cd treatment, we detected O2− and H2O2 accumulation were detected and the activities of their corresponding catabolic enzymes (SOD and CAT) in new leaves. The concentrations of both O2− and H2O2 in P. aizoon and K. blossfeldiana did not increase under Cd treatment (Fig. 2a, b), indicating that ROS do not cause oxidative damage to these two plants under 50 mg·kg−1 soil Cd. Interestingly, it was found that SOD activity in both P. aizoon and K. blossfeldiana was significantly induced by Cd treatment (0.01 < p < 0.05 or 0.001 < p < 0.01) (Fig. 2c). Similarly, the CAT activity markedly increased in P. aizoon (0.001 < p < 0.01), whereas it tended to increase in K. blossfeldiana under the Cd treatment (Fig. 2d).
Figure 2.
Reactive oxygen species and antioxidant enzyme activities of P. aizoon and K. blossfeldiana under control and Cd treatment (50 mg·kg−1) conditions. (a) Superoxide anion (O2−) content. (b) H2O2 content. (c) SOD activity. (d) CAT activity. Data represent means ± standard deviations (n = 3). * and ** indicate significant differences between two groups at 0.01 < p < 0.05 and 0.001 < p < 0.01, respectively. FW: fresh weight.
Cd uptake and transfer
-
Cd concentrations in the leaves, stems, and roots of P. aizoon were 212.0, 104.0, and 83.5 mg·kg−1, and of K. blossfeldiana were 101.5, 53.7, and 125.0 mg·kg−1, respectively (Fig. 3a). Cd concentration in the leaves of P. aizoon was significantly (p < 0.05) greater than those in the stems and roots, whereas the Cd concentration in the roots of K. blossfeldiana was markedly (p < 0.05) greater than those in the leaves and stems (Fig. 3a). In addition, it was found that the Cd concentrations in the leaves and stems of P. aizoon were 2.1 and 1.9 times higher than those in K. blossfeldiana, respectively, (Fig. 3a).
Figure 3.
Cd accumulation indices of P. aizoon and K. blossfeldiana under Cd treatment (50 mg·kg−1). (a) Cd concentrations in leaves, stems, and roots. (b) Bioconcentration factor (BCF) of three plant parts. (c) Translocation factor (TF) of the leaf and stem. (d) Cd accumulation contents in individual plants and their aboveground parts. (a) and (b) The bars distinguished by different letters (a, b, α, or β) significantly vary among the tissues of P. aizoon or K. blossfeldiana at a significance level of 0.05. (a)−(c) The dashed lines indicate the threshold values of Cd hyperaccumulators. Data represent means ± standard deviations (n = 3). *, **, and *** represent significant differences between two groups at 0.01 < p < 0.05, 0.001 < p < 0.01, and p < 0.001 levels, respectively. DW: dry weight.
The mean Cd BCF values in the leaves, stems, and roots of P. aizoon were 4.24, 2.08, and 1.67, respectively, whereas those of K. blossfeldiana were 2.03, 1.07, and 2.50, respectively (Fig. 3b). The differences in Cd BCFs between the two plants were similar to those in the Cd concentrations (Fig. 3b), as the plants were planted in the same soil. The mean Cd TFs in the leaves and stems of P. aizoon were 2.54 and 1.25, respectively, whereas those of K. blossfeldiana were 0.84 and 0.44, respectively (Fig. 3c). For each species, the TF in the leaves was significantly (p < 0.001 or 0.01 < p < 0.05) greater than that in the stems (Fig. 3c). The TFs of both the leaves and stems of P. aizoon were significantly (p < 0.001 or 0.001 < p < 0.01) greater than those of K. blossfeldiana (Fig. 3c).
Based on plant biomasses and Cd concentrations, Cd accumulation in the entire individual plant and the aboveground parts of each species was assessed. The mean Cd contents in individual plant of P. aizoon and K. blossfeldiana were 2.01 and 1.31 mg, and in their aboveground parts were 1.76 and 1.15 mg, respectively (Fig. 3d). Cd accumulation in P. aizoon, whether in the whole plant or the aboveground part, was higher than that in K. blossfeldiana (0.01 < p < 0.05) (Fig. 3d). The proportions of the Cd content in the aboveground parts of P. aizoon and K. blossfeldiana were similar (87%–88%) (Fig. 3d).
TPR results
-
To evaluate the phytoextraction potential of Cd-contaminated soils, we estimated the TPR values of P. aizoon and K. blossfeldiana. The mean TPRs of P. aizoon and K. blossfeldiana grown in 50 mg·kg−1 Cd-contaminated soil for 3 months were 0.33% and 0.65%, respectively (Fig. 4). Notably, it was found that the TPR of K. blossfeldiana was 1.97 times greater than that of P. aizoon under the same soil condition and growth period (Fig. 4).
-
Heavy metal phytoextraction efficiency largely depends on high tolerance and accumulation capacities of plants; thus, numerous studies have focused on screening and identifying high-heavy metal-accumulating species, especially hyperaccumulators[24]. In Crassulaceae, Sedum alfredii, and S. plumbizincicola have been identified as zinc/Cd hyperaccumulators[25], and their physiological and molecular mechanisms underlying Cd tolerance and uptake have attracted much interest[26−29]. These findings have prompted scientists to screen novel Cd accumulators or hyperacccumulators from other Crassulaceae species. For example, Wu et al. analysed the Cd accumulation characteristics of 11 generalized Sedum species[30], which included Sedum aizoon (namely, P. aizoon in this study). The results showed that P. aizoon could tolerate 100 mg·kg−1 but succumb to 200 or 400 mg·kg−1 soil Cd. Additionally, both the BCF in the aboveground part and the TF were < 1[30], which value is the threshold for determining whether a species belongs to hyperaccumulators[17]. However, in another study, both the shoot BCF and TF of P. aizoon were > 1 when treated by low Cd concentrations[31]. Interestingly, P. aizoon used in this study met all the threshold values (i.e., aboveground part Cd concentration > 100 mg·kg−1, BCF > 1, and TF > 1) for Cd hyperaccumulators under 50 mg·kg−1 soil Cd treatment (Fig. 3), indicating that it may be a potential Cd hyperaccumulator. For K. blossfeldiana, the results of Cd accumulation characteristics (Fig. 3) indicate it can be considered a high-Cd-accumulating species, which is superior to the Cd accumulation capacity of K. blossfeldiana reported in a recent study[32]. The distinct results from different studies may be attributed to multiple factors, including plant genotype[33], soil property[34], and Cd treatment time. Overall, both P. aizoon and K. blossfeldiana genotypes used in this study are high-Cd-accumulating species, which can thus be considered for the phytoextraction of Cd-polluted soils.
Additionally, Phedimus aizoon has often been used as a medicinal vegetable by some people in China, as it contains many medicinal ingredients[35]. The present results thus suggest that appropriate attention should be paid to the safety of P. aizoon consumption because of the risk of Cd intake.
Cd tolerance mechanism in P. aizoon and K. blossfeldiana
-
To improve phytoextraction efficiency via genetic engineering or other strengthening measures, understanding the physiological and molecular mechanisms by which plants tolerate and accumulate heavy metals is important[33,36]. In this study, although P. aizoon and K. blossfeldiana accumulated a large amount of Cd in their leaves, their physiological activities (e.g., chlorophyll synthesis) were not harmed. These results suggest that these two plants provide effective protection strategies against Cd stress.
Previous studies have demonstrated that plants usually involve a trade-off between the accumulation and elimination of ROS under Cd stress[37,38]. The accumulation of ROS (e.g., O2−, H2O2, and •OH) can cause damage to DNA, proteins, and lipids when stress occurs[39]. However, plants have evolved effective mechanisms to regulate ROS homeostasis[40]. Antioxidant systems, including antioxidant enzymes (e.g., SOD, CAT, and peroxidase) and nonenzymatic antioxidants are responsible for adjusting ROS homeostasis[41]. Specifically, SOD catalyzes the dismutation of O2− to produce O2 and H2O2 in cells, whereas H2O2 can be further catalyzed to decompose into O2 and H2O by CAT[41]. In this study, the induced activities of SOD and CAT and the steady concentrations of O2− and H2O2 fully illustrate that P. aizoon and K. blossfeldiana can effectively start the antioxidant system to control ROS accumulation to avoid oxidative damage.
Recently, S. plumbizincicola and S. alfredii have gradually become model species for investigating the mechanisms of Cd tolerance and accumulation in plants[26,28,29,42−44]. Several important genes have been found to participate in Cd detoxification or transport in them. For example, Liu et al. reported that the tonoplast-localized SpHMA3 protein could transport Cd into vacuoles for compartmentalization to reduce Cd toxicity in the shoots of S. plumbizincicola[43]. Zhao et al. reported that the SpHMA1 protein can export Cd from chloroplasts to protect against photosynthesis[29]. Most of these genes have also been reported in other species with similar functions[29,45,46]. To date, owing to the lack of whole-genome information, few unique tolerance and accumulation mechanisms have been identified in S. plumbizincicola, which has higher Cd tolerance and accumulation capacities than most Cd hyperaccumulators[25]. Crassulaceae species have unique crassulacean acid metabolism pathways; thus, it is of interest to explore whether these metabolic processes contribute to Cd detoxification in high-Cd-accumulating Crassulaceae species. For P. aizoon and K. blossfeldiana, more work should be conducted to analyse their physiological and molecular mechanisms in response to Cd.
Phytoextraction potential of P. aizoon and K. blossfeldiana for Cd-polluted soils
-
Heavy metal accumulators or hyperaccumulators are the preferred resources for phytoextraction. However, studies have also indicated that plant biomass and growth rate are important for phytoextraction efficiency[11,47]. Indeed, for many reported heavy metal accumulators or hyperaccumulators, their actual phytoextraction potential has not been well analysed by combining factors such as growth rate or biomass accumulation. In this study, although P. aizoon and K. blossfeldiana presented high Cd accumulation capacities, their growth rates were relatively mediocre. For example, the dry weight biomasses of 3-month-old P. aizoon and K. blossfeldiana were approximately equal to those of 2-month-old turnip, rape, and Phytolacca americana reported previously[23] under similar soil conditions. However, the high biomass of individual plants of certain species does not mean that higher total biomass yields can be harvested in a unit area. The shape and size of different plants determine their optimum planting densities in the field, thus affecting the total biomass yields in a unit area. Generally, upright-type plants (e.g., K. blossfeldiana) can have higher optimum planting densities than scatted-type species (e.g., P. aizoon). This can increase the total biomass yield of upright-type plants per unit area, which is confirmed by the present estimation results for P. aizoon and K. blossfeldiana. On the whole, we believe that the ultimate phytoextraction efficiency will depend on the capacity of a plant to accumulate pollutants and biomass yield, as well as planting density determined by plant shape and size.
In this study, the TPR index was introduced, which integrates all of the above mentioned factors, to evaluate the Cd phytoextraction potentials of P. aizoon and K. blossfeldiana. The results revealed the Cd extraction rates by P. aizoon and K. blossfeldiana from the soil layer at a depth of approximately 25 cm (root length) per unit area under the appropriate planting density for 3 months of growth. Interestingly, the TPR value of K. blossfeldiana was markedly greater than that of P. aizoon, although Cd accumulation in the aboveground part of individual P. aizoon plant was much greater than that of individual K. blossfeldiana plant. This finding highlights the importance of plant shape on phytoextraction rates when screening and breeding hyperaccumulating plant resources. The TPR index provides a bridge between experimental research (e.g., pot experiments) and actual phytoextraction efficiency in the field. However, it is necessary to verify the reliability of this theory in combination with practical phytoextraction work in the future.
-
Both P. aizoon and K. blossfeldiana genotypes used in this study tolerated 50 mg·kg−1 soil Cd stress and the antioxidant system might be involved in Cd detoxification. The results of the Cd concentration, BCF, and TF analyses revealed that P. aizoon is a potential Cd hyperaccumulator, whereas K. blossfeldiana is a high-Cd-accumulating species. Considering that the ultimate phytoextraction efficiency is affected by Cd accumulation ability of plants, plant biomass, and plant shape and size, the integrated index TPR was introduced to compare the potential phytoextraction rates between P. aizoon and K. blossfeldiana. Interestingly, the TPR value of K. blossfeldiana was approximately two times greater than that of P. aizoon, although Cd accumulation in the aboveground part of individual P. aizoon plant were significantly higher than that of individual K. blossfeldiana plant. This study offers new resources for phytoextraction of Cd-contaminated soils and a novel theoretical method to evaluate phytoextraction efficiency.
This work was financially supported by the Plateau Characteristic Agriculture Science and Technology Plan of Yunnan Province (202302AE090023), and the International Joint Laboratory for Mountain Agricultural Ecosystems of Yunnan Province (202303AP140001).
-
The authors confirm contribution to the paper as follows: study conception and design, data analysis, draft manuscript preparation, manuscript revision: Li X, Li Y, Huang X; experiments conduction: Li Y, Huang X, Karel NNJ, Tang H, Hu N. All authors reviewed the results and approved the final version of the manuscript.
-
The authors declare that they have no conflict of interest.
-
# Authors contributed equally: Xumei Huang, Yanshuang Li
- Copyright: © 2024 by the author(s). Published by Maximum Academic Press, Fayetteville, GA. This article is an open access article distributed under Creative Commons Attribution License (CC BY 4.0), visit https://creativecommons.org/licenses/by/4.0/.
-
About this article
Cite this article
Huang X, Li Y, Karel NNJ, Tang H, Hu N, et al. 2024. Theoretical phytoextraction rates evaluating the application potential of two Cd accumulators from Crassulaceae for cleaning Cd-contaminated farmland. Circular Agricultural Systems 4: e018 doi: 10.48130/cas-0024-0018
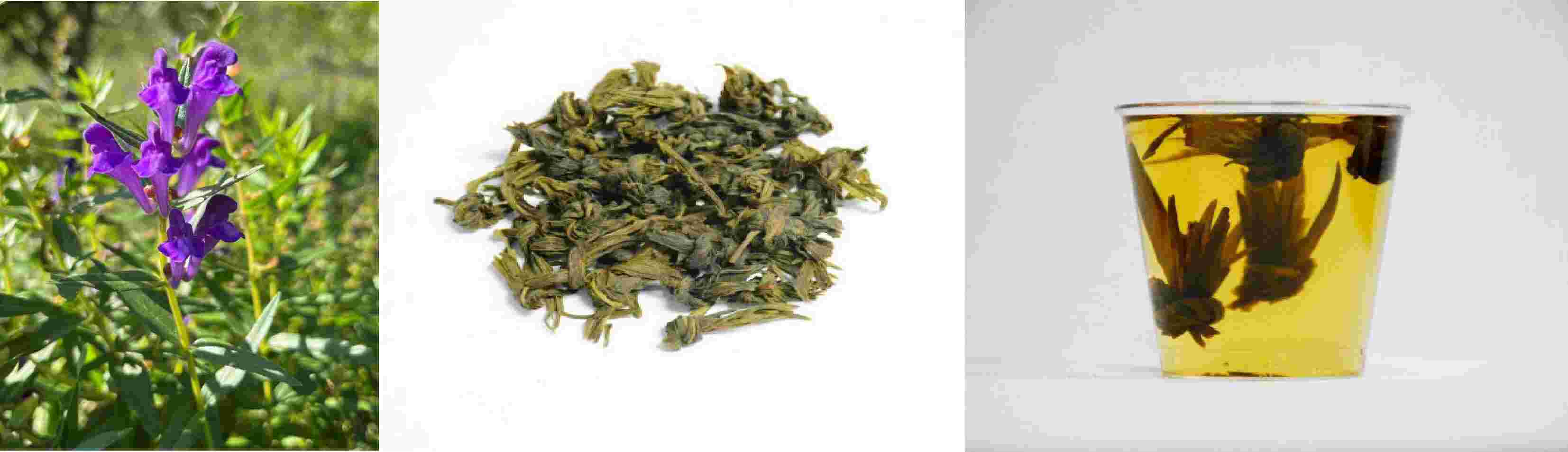