-
Tea contains abundant phenolic compounds. As the main secondary metabolites of tea plants, they play an essential role in determining tea quality and health efficacy. Tea polyphenols are potentially beneficial to human health due to their antioxidant capacity, electrophilic ion scavenging ability, and metal ion chelation ability[1]. The amounts of tea polyphenols accumulated in different tissues and organs of tea plants are significantly distinct. Tea polyphenols are mainly accumulated in tender shoots[2]. With the development and maturation of tea leaves, the content of gallocatechin (GC), catechin (C), and epicatechin (EC) changes little, epigallocatechin (EGC) content gradually elevates, and the content of gallate catechin epigallocatechin gallate (EGCG) and epicatechin gallate (ECG) slowly descends[3].
At the growth stage, the order of total polyphenol content in multiple organs of tea plants is leaf > stem > root[4]. Deng & Ashihara[5] studied the relative concentration and quantity of theanine in various parts of tea seedlings growing for 27, 106, and 205 d and biosynthetic activity. The results show that caffeine and its biosynthetic activity merely occur in leaves and stems, while theanine is widely distributed in all organs, especially the roots, the major organ synthesizing and transferring theanine to the leaves. Lu et al.[6] explored the regulatory effect of light signal on the biosynthesis and accumulation of tea polyphenols and discovered that the accumulation patterns of phenolic acids, flavan-3-ols, and flavonols in tea seedlings were inconsistent during the de-etiolation process. The effect of light intensity on catechin biosynthesis is mediated by plant photosynthetic capacity[7]. Increasing CO2 concentration can enhance the photosynthetic capacity and the growth of tea seedlings. It is speculated that soluble nutrients and functional components in the leaf surfaces of tea seedlings will increase with atmospheric CO2 concentration, thus promoting tea quality and taste[4]. When the temperature difference between day and night is 10 °C, the content of soluble sugar, tea polyphenols, and catechins of tea plants is the lowest, and caffeine and amino acids are copious, which is suitable for the protection and cultivation of tea seedlings[8]. Under low phosphorus levels, the inoculation of Serendipita indica can stimulate phosphorus acquisition and the growth of tea seedlings by increasing the expressions of cytokinin, indole acetic acid, phosphate transporter 1, and phosphate transporter 4[9]. The excessive application of phosphate fertilizer results in an elevated phosphorus content in the soil, which hampers the accumulation of polyphenols while promoting the accumulation of certain anthocyanins[10]. The above results manifest that environmental factors can profoundly influence the growth of tea seedlings.
Chalcone synthase (CsCHS), chalcone isomerase (CsCHI), and flavanone 3-hydroxylase (CsF3H) are the upstream genes of flavonoid synthesis pathway and the key regulatory genes of flavonoid content[11,12]. The expression of CsF3H is positively correlated with the syntheses of catechins and anthocyanins and is essential for the hydroxylation of the C ring[13−15]. Flavonoid 3'-hydroxylase (CsF3'H) is involved in the biosyntheses of B-3' and 4'-dihydroxyflavone, and the expression level is positively correlated with the accumulation patterns of 3' and 4'-catechin, 3,' 4,' and 5'-catechin, and flavan3-ol in tea leaves[16,17]. Leucoanthocyanidin can be hydroxylated by CsF3'H on the B-ring, and CsF3'H gene expression is significantly correlated with soluble PAs concentration[18,19]. Experiments on the heterologous expressions of flavonoid 3' and 5'-hydroxylase (CsF3'5'H) in yeasts have shown that the expression of CsF3'5'H is closely related to the accumulation of flavonoid end products in tea buds and leaves[20]. Functional single nucleotide polymorphism alleles within CsF3'5'H control the ratio of di/tri-hydroxylated catechins and catechin content[21]. Flavonol accumulation is consistent with the expression of flavonol synthases (CsFLS), responsible for the biosynthesis of flavonols with dihydroflavonol as substrate[22,23]. The accumulation of C-type PAs has a strong positive correlation with the expression of dihydroflavonol 4-reductase (CsDFR)[24]. Anthocyanidin reductase (CsANR) expression level is significantly correlated with soluble PA concentration[19]. In situ hybridization experiments reveal that genes related to catechin synthesis CsDFR1, CsDFR2, leucocyanidins reductase (CsLAR), and CsANR1 are significantly expressed in primordial and young leaves[25]. The exogenous overexpression of CsFLSs in tobacco promotes the biosynthesis of flavonols and inhibits anthocyanin accumulation. The overexpression of CsDFRs can down-regulate the expression of endogenous CsFLS and accelerate anthocyanin synthesis, suggesting that CsFLS and CsDFRs regulate the accumulation of flavonols and anthocyanins by competing for common substrate dihydroflavonol[26]. The transcript levels of LAR and ANR correlate with catechin and epicatechin, which are proanthocyanidin precursors[27]. The catechin carbocation in CsANRa transgenic tobacco increases significantly and dynamically interacts with EC-typeleucocyanidin. (−)-epicatechin or (+)-catechin is incubated with epicatechin carbocation in CsANRa transgenic flower extracts to form dimeric procyanidin B1 or B2[28,29] . Yao et al.[30] adopted different acyl receptor donors to detect the substrate diversity of co-expressed recombinant gallate catechin synthetase (CsSCPL4 and CsSCPL5). The expression of SCPL11-1 was higher in Camellia ptilophylla, a natural theobromine-rich tea, which may be the main cause of gallocatechin gallate (GCG) enrichment[31]. The UPLC-MS/MS results show that the products of co-expressed CsSCPL4 and CsSCPL5 include EGCG and ECG, without C or GC.
This paper explores the differences in flavonoid accumulation at multiple development stages of two species of tea seedlings, 'Mengku Dayecha' (MKDY) and 'Huangkui' (HK), using the metabolomics research method. This systematic study can provide a theoretical foundation for the biological research of tea plants.
-
'MKDY' (C. sinensis var. assamica, CSA) is an arbor-type tea plant with a tall crown and rich in substances, while 'HK' (C. sinensis var. sinensis, CSS) is a shrub type with golden yellow buds and leaves, rich in amino acids, lutein, and carotene. The tea seeds of 'MKDY' and 'HK' planted in culture trays were cultivated in a greenhouse of Anhui Agricultural University (Hefei, China, 31.52° N and 117.14° E) and watered every three to five days. The development of 'MKDY' seedlings was divided into five stages (S1−S5), and that of 'HK' seedlings was separated into four stages (S1−S4). The leaf colors of 'HK' seedlings at S4 included dark green (D), light green (L), and yellow-white (W) (Fig. 1a & b). In this study, the appearance characteristics of tea seedlings were visually documented and recorded at each stage of growth and development. Additionally, a random selection of 50 tea seedlings was made at each growth stage to measure both the aboveground and underground lengths. The root tips (1 cm), hypocotyl parts (from cotyledon to radicle, about 2 cm), epicotyl segments (between the cotyledon initiation and the first true leaf), and shoots (bud and leaf) of tea seedlings at each stage were collected, immediately frozen with liquid nitrogen, and stored at −80 °C.
Figure 1.
Growth phenotype and physiological indexes of tea seedlings in different stages. (a), (b) Pictures of tea seedlings of 'MKDY' and 'HK' at different growth stages, and the parts of experimental materials taken from different tissues are marked in the figures. (c), (d) Plant height and root length of 'MKDY' tea seedlings. (e), (f) Plant height and root length of 'HK' tea seedlings in different stages.
Extraction and determination of flavonoids
Flavonoid extraction
-
The freeze-dried materials were ground into powders with a mortar and pestle at room temperature, filtered by an iron sieve with a diameter of 200 mesh, and then mixed into a 10 mL centrifuge tube. The tube was sealed with a sealing film and stored at −20 °C for the subsequent experiments.
The tea samples were taken from the refrigerator. After restored to normal temperature, 0.025 ± 0.0001 g of tea samples were accurately weighed and transferred to a 2 mL centrifuge tube equipped with two white zirconium beads in advance. Then, 1 mL of 80% methanol was absorbed into the centrifuge tube using a pipette gun. Ultrasonic extraction was performed on the tube with an ultrasonic instrument at a low temperature and a frequency of 20 Hz/s for 10 min. After extraction, the tube was centrifuged at 13,000 rpm and 15 °C for 10 min. The supernatant was sucked into a 2 mL centrifuge tube as much as possible. The extraction steps were repeated on precipitated polyphenols, and the supernatant was added into the tube. Finally, the polyphenol extract was centrifuged, and the supernatant was transferred to a new centrifuge tube for testing. Due to the dark color of the shoot extract, trichloromethane and Watsons water were incorporated for determination. The volume ratio of extract, trichloromethane, and Watsons water was 1:1:1.
Flavonoid determination
-
The detection method of total polyphenols, total flavanols, PAs, and total flavanols was based on Fu et al.[32]. Three biological replicates were set up and each sample was measured three times.
Vanillin and 1 mol/L Folin−Ciocalteu were purchased from Shanghai Yuanye Bio-Technology Co., Ltd. (Shanghai, China). The other reagents and medicines came from Sinopharm Chemical Reagent Co., Ltd. (Shanghai, China).
Metabolic analysis of flavonoids
-
The diluted tea polyphenol extract was centrifuged at 13,000 rpm/min for 10 min. Then, 50 μL of the supernatant was sucked into a sample bottle. The time-of-flight mass spectrometry (TOF-MS) and ultra-performance liquid chromatography-triple quadrupole mass spectrometry (UPLC-QQQ-MS/MS) were detected by referring to Jiang et al.[33]. The deionized water used in the experiment was obtained from the MilliQ direct 8 pure water instruments (France) for TOF-MS and UPLC-QQQ-MS/MS. High-performance liquid chromatography grade acetic acid, acetonitrile, and methanol were purchased from TEDIA Co, Ltd. (Fairfield, OH, USA).
qRT-PCR analysis of flavonoid pathway genes
-
This study consulted Wang et al.[19] about the two-step real-time fluorescent quantitative PCR detection method. The data was represented by the average of three replicates. The enzymes and primers used in this test came from Shanghai Yisheng Biotechnology Co., Ltd (Shanghai, China).
Data analysis
-
GraphPad Prism 9 was employed to depict the quadrant plots of physiological indicators of tea seedlings. Multiple reaction monitoring (MRM) mass spectrometry data were integrated using Agilent Mass Hunter Qualitative Analysis B.07.00 software. Microsoft Excel 2019 was used to calculate relative peak area values and generate heat maps. One-way ANOVA and least significant difference (LSD) were performed using IBM SPSS Statistics 24 to determine the mean ± standard deviation of multiple biological replicates and the significance. The Pearson correlation analysis and PCA analysis were performed using SPSS, version 22. Other data analysis was carried out in Microsoft Excel 2019.
-
During the growth and development of tea seedlings, this study photographed and recorded the appearance characteristics of tea seedlings in each stage and measured the lengths of aboveground and underground parts (Fig. 1).
The physiological state of 'MKDY' seedlings changed continuously in germination (Fig. 1a). After a stage of soil cultivation, the roots began to develop and grew white, tender, and strong root tips (S1). Then, the buds and leaves spread out (S2), and the epicotyl segments became distinguishable (S3). With the maturity of tea seedlings, the stems became longer and thicker, and the leaves proliferated and darkened (S4 and S5).
It can be found that the roots of tea seedlings develop first. When the roots grow to a specific degree, the germination begins. The root development slows down during epicotyl growth. The roots mature and become yellow with plant development. Meanwhile, the buds and leaves continuously stretch and ripen. The plant heights and root lengths of tea seedlings at different germination stages verify this view (Fig. 1c & d). In S2, the aboveground part sprouted, followed by the buds and leaves. In S2−S5, the plants grew fast, from 2.56 to 8.38 cm, with significant differences among stages. By comparison, the root elongation was slow, from 7.53 to 10.65 cm. Although the aboveground part starts germination late, the growth rate is faster than that of the roots. However, the latter is more stable.
According to the phenotype diagram (Fig. 1b) and physiological indexes (Fig. 1e & f) of 'HK' seedlings, the growth patterns of aboveground and underground parts are consistent with those of 'MKDY.' There is no significant difference in root lengths in S1 and S2, while the roots grow significantly in S3 and S4. It is worth mentioning that the difference in the root lengths of tea seedlings with different leaf colors is minor in S4. The plant height in S3 is significantly different from that in S4D and S4L and almost the same as in S4W. The plant height of tea seedlings in S4W is obviously lower than that of tea seedlings with different leaf colors in the same stage. In conclusion, plant height and root length are positively correlated with maturity.
Differences in flavonoid accumulation among development stages of tea seedlings
-
To investigate the metabolic characteristics of flavonoids during the growth and development of tea seedlings, this study collected the root tips, hypocotyl parts, epicotyl segments, and tender shoots of two varieties of tea seedlings at multiple development stages. The content of total polyphenols, flavanols, PAs, and flavonols in each organ was quantitatively detected using an ultraviolet spectrophotometer (Figs 2 & 3).
Figure 2.
Flavonoids accumulation pattern of 'MKDY' tea seedlings at different developmental stages. (a)−(d) Were the accumulation of total polyphenols, flavanols, PAs and flavonols in shoots, epicotyls, hypocotyls and root tips, respectively. DW: dry weight.
Figure 3.
Flavonoids accumulation pattern of 'HK' tea seedlings at different developmental stages. (a)−(d) Were the accumulation of total polyphenols, flavanols, PAs and flavonols in shoots, epicotyls, hypocotyls and root tips, respectively. DW: dry weight.
In shoots, the accumulation patterns of total polyphenols and total flavanols in 'MKDY' (Fig. 2a) are the same. The content of total polyphenols and total flavanols rises considerably in the development stage, positively correlated with the maturity of tea seedlings. PAs content decreases in the growth stage. The content of total flavonols fluctuates and increases in the development stage. The accumulation of total polyphenols and flavanols in 'HK' (Fig. 3a) is consistent with that in 'MKDY'. PAs content decreases in the growth stage, and the difference is significant in various growth stages. Flavonol content decreases first and then increases.
In the epicotyl segments, the content of flavonoids in 'MKDY' (Fig. 2b) decreases with development. Except for total polyphenol content, there is no significant difference in flavonoid content at multiple growth stages. The results of 'HK' (Fig. 3b) are similar. The accumulation of flavonoids presents a downward trend in general, with significant differences among growth stages.
In the hypocotyl parts, the accumulation patterns of total polyphenols, flavanols, and PAs in 'MKDY'(Fig. 2c) during S1−S4 are not obvious. Flavonol content declines obviously in S1−S3 and elevates slightly in S4. The accumulation of total polyphenols, flavanols, and PAs in 'HK' (Fig. 3c) decreases in each stage, while the flavonol content changes mildly.
In the root tips, the content of total polyphenols, flavanols, and flavonols of 'MKDY' (Fig. 2d) gradually increases during S1-S4, and flavonol content has no significant change overall. In HK (Fig. 3d), the accumulation of total polyphenols and flavanols has no apparent pattern during S1−S4. With the growth of tea seedlings, the accumulation of PAs enhances, and the accumulation of total flavonols increases first and then descends. In the 'HK' chromogenic technology results (Fig. 3), the content of flavonoids in S4L is higher than that in S4D, and the accumulation of flavonoids in S4W has no obvious pattern.
In conclusion, there is no significant difference in the accumulation patterns of polyphenols in the same organ of tea seedlings among growth and development processes.
Difference in flavonoid accumulation among tea seeding organs
-
In order to explore differences in accumulated flavonoids among organs, this study selected tea seedlings in S3 and S4 when the 'MKDY' seedlings were fully developed, and S4D and S4L in S4 of 'HK' seedlings to detect the accumulation of flavonoids in different organs (Fig. 4).
Figure 4.
Accumulation pattern of flavonoids in different tissues. (a), (b) Accumulation of flavonoids in different tissues at S2 and S3 stages of 'MKDY' tea seedlings. (c), (d) Accumulation of flavonoids in different tissues during S4D and S4L stages of 'HK' tea seedlings. DW: dry weight.
The chromogenic technology results show that the total polyphenols, flavanols, and flavonols in the two varieties of tea seedlings are enriched in the epicotyl segments and shoots. The accumulation in each organ decreases from the aboveground part to the underground part. In the S3 and S4 stages of 'MKDY' (Fig. 4a & b), the total polyphenol content is 1.8 and 2.8 times the flavanol content in shoots, respectively. The accumulation in the underground part is less. PAs are mostly enriched in epicotyl segments and gradually decrease to root tips. The epicotyl content of PAs in S4 is 2.8 times that in shoots. No significant difference exists in the accumulation of flavonols in the aboveground and underground parts in S3. Flavonol content in the shoots in S4 is about three times that in the other three organs. The accumulation patterns of flavonoids in the S4D and S4L stages of 'HK' (Fig. 4c & d) are basically consistent with those of 'MKDY.' The content of total polyphenols and flavanols in shoots is about 15% higher than those in epicotyl segments and three times higher than those in hypocotyl parts and root tips. PAs are concentrated in the epicotyl segments, 5.4 times that in the shoots. Unlike 'MKDY,' 'HK' has the least PAs in the shoots. The accumulation of flavonols in shoots is about twice in hypocotyl parts and root tips, and in S4D seedlings, the content in epicotyl segments decreases more than in the shoots.
Since the accumulation pattern of flavonoids varies greatly with organs, this study carried out a mass spectrometry analysis. Four organs in the S2 and S4 stages of 'MKDY' were selected. The shoots and root tips of the S4L stage of 'HK' were taken as the object of reference. The UPLC-QQQ-MS/MS and the TOF-MS system were adopted to qualitatively detect 'MKDY' and 'HK,' respectively (Figs 5 & 6).
Figure 5.
Qualitative and quantitative analysis of flavonoids in 'MKDY' tea seedlings. (a) Total ion chromatogram of flavonoids in S4 stage. (b) Heat map of flavonoids in S2 and S4 stages. (c) PCA analysis of flavonoids in 'MKDY' tea seedlings.
Figure 6.
Qualitative and quantitative analysis of flavonoids in 'HK' tea seedlings. (a) - (c) Ion chromatogram of flavanols, PAs, and flavonols in shoot and root of S4L stage, respectively. The green and gray peak lines represent the shoot and root, respectively. (d) Heat map of flavonoids in S4L stage.
According to the mass chromatogram of 'MKDY' seedlings in S4 (Fig. 5a) and 'HK' seedlings in S4L (Fig. 6a−c), the amounts of flavanols, PAs, and flavonols accumulated in aboveground and underground parts are significantly different (Figs 5b & 6d). Among flavanols, simple catechin C and EC are contained in all organs. The content in 'MKDY' shoots is remarkably higher than in 'MKDY' root tips. For 'HK,' the content of C in shoots is slightly higher than in root tips, and EC content in root tips is about 1.7 times that in shoots. The gallate catechins, including ECG and EGCG, are mainly concentrated in the aboveground part of the two varieties and barely in the underground part. In general, the overall accumulation of flavanols in the aboveground part is higher than that in the underground part, consistent with the chromogenic technology results.
In 'MKDY' seedlings (Fig. 5b), PAs are highly accumulated in the hypocotyl parts at the S2 stage, and the content of PA B2 and PAC1 in the shoots is significantly higher than in the root tips. EC-EGC and EGC-EGCG are mainly accumulated in shoots at the S2 stage and the epicotyl segments at the S3 stage, except for the root tips. In 'HK' seedlings (Fig. 6d), PA B2 and PA C1 are enriched in root tips and basically do not exist in shoots, while EC-EGC and EGC-EGCG are merely accumulated in shoots.
In 'MKDY' seedlings in the S2 stage (Fig. 5b), the content of flavonol glycosides, such as K-3-O-galactosylrutinoside (K-3-gal-r), K-3-O-glucosylrutinoside (K-3-glu-r), Q-3-O-galactosylrutinoside (Q-3-gal-r), Q-3-O-glucosylrutinoside (Q-3-glu-r), and M-3-O-glucosylrutinoside (M-3-glu-r), is significantly higher in shoots than that in the underground part. In the S4 stage, massive M-3-glu-r accumulates in shoots. K-3-gal-r, Q-3-gal-r, and Q-3-glu-r are enriched in epicotyl segments. Moreover, these four substances only exist in aboveground organs. M-3-O-galactosylrutinoside (M-3-gal-r) is not detected in this experiment. In 'HK' seedlings (Fig. 6d), the accumulation pattern of flavonol glycosides mentioned above is similar to that in 'MKDY.' Abundant flavonol glycoside K-rha-r accumulates in the root tips of both varieties. In general, the accumulation patterns of flavonols in the two varieties of tea seedlings are similar, and the content of flavonols in the aboveground part is higher than that in the underground part.
The results of PCA analysis revealed that the accumulation patterns of flavonoids in aboveground (shoot and epicotyl) and underground parts (root and hypocotyl) was clustered together separately (Fig. 5c). The aboveground part mainly accumulates B-ring di/tri-hydroxyl catechins (EC, C, GC, and EGC), gallate catechins monomers (ECG and EGCG), and polymers, while the underground part amasses B-ring di-hydroxyl catechins (EC and C) and special flavonol glycosides (K-rha-r).
Expression analysis of flavonoid pathway genes
-
In order to verify the detection results of the mass spectrum, 'MKDY' seedlings in S3 were used to measure the expression levels of flavonoid synthesis pathway genes. The qRT-PCR results show that CsF3'5'Ha, which controls B-ring tri-hydroxyl catechins synthesis, and CsSCPL4-1 and CsSCPL5,which control gallate catechins, are highly expressed in the aboveground part, especially the shoots, and few in the underground part (Fig. 7b). CsANRa is expressed in all organs, and especially, the relative expression in root tips is 1.8 times in shoots. The relative expressions of other genes in the aboveground part are significantly higher than those in the underground part. The relative expressions of CsCHSa, CsCHIa, CsF3Ha, CsF3'H, CsFLSa, and CsDFR are consistent in the four organs, with the highest in the shoots and the lowest in the hypocotyl parts. CsANSa is highly expressed in the epicotyl segments. CsLARa has the highest relative expression in the shoots and seldom be expressed in the underground part.
-
Tea polyphenols have been extensively applied in medicine, food, cosmetics, and other fields. Their use value has been demonstrated. More value is under exploration, especially in EGCG research. Tracing the accumulation of polyphenols in tea plants can directly identify the concentration of tea polyphenols, laying a foundation for the extraction and utilization of tea polyphenols. It is of great significance. Most studies focused on the accumulation of flavonoids in adult tea plants, and few were on the growth and development of tea seedlings. Some studies revealed that it was difficult to detect gallate catechins in the roots of tea seedlings growing for 25 to 90 d. However, gallate catechin EGCG and ECG can be detected in the roots developed for ten days[34]. With current knowledge and technologies, this is almost impossible. It is assumed that the substance accumulated in developing roots is not EGCG but PAs with similar retention time and absorption peaks. In this study, EGCG, ECG, and other gallate catechins accumulate abundantly in shoots. In contrast, they are almost undetectable in the roots. According to the differences in the accumulation of flavonoids among organs of the two kinds of tea seedlings (Fig. 4), total polyphenols, flavanols, and flavonols are enriched in the epicotyl segments and shoots, and the accumulation decreases from the aboveground part to the underground part. It is consistent with the finding that the order of total catechin content in tea plants at each growth stage is leaf > stem > root[4]. PAs are concentrated in the epicotyl organs, which is different from the well-known conclusion that PAs are accumulated in stems and roots. PAs content in tea plant roots is up to 8%[35]. It indicates that the accumulation pattern of PAs in tea seedlings differs from that in mature tea plants.
The results of the mass spectrum (Figs 5 & 6) show that the types of flavonoids accumulated in the aboveground and underground parts are extremely diverse. The aboveground part mainly accumulates B-ring di/tri-hydroxyl catechins, gallate catechins monomers, and polymers, while the underground part only accumulates B-ring di-hydroxyl catechins and special flavonol glycosides. PA B2 and PA C1 are accumulated in the root tips of 'HK' tea seedlings and the hypocotyl parts of 'MKDY' tea seedlings. This is because the hypocotyl parts are a relatively mature organ formed during the growth and development of tea seedlings. Many studies have similar results. For example, the results of UPLC-QQQ-MS/MS analysis manifest that the content of non-gallate catechin and oligopolymeric PAs significantly lowers in the upper stem and increases in the lower stem. C, EC, and oligomeric PAs are accumulated the most, and flavonoid glycosides are abundant and higher in young leaves[36,37].
Furthermore, the qRT-PCR analysis results of flavonoid pathway genes can interpret the results of the mass spectrum (Fig. 7b). Except for CsANRa, the expression level of CsANRa in the underground part is considerably higher than that in the aboveground part, and the expression levels of other genes in the above part are significantly higher than that in the underground part. It agrees that the total polyphenol and flavanol content is apparently higher in the aboveground part than in the underground part. CsF3'H and CsF3'5'H are important enzymes for the hydroxylation of the B-ring of flavonoids and can catalyze the formation of corresponding 3',4' or 3',4',5' -hydroxylated flavonoid compounds[15−17,20,38]. The expression level of CsF3'5'H is high in the aboveground part, especially in the shoots, but barely in the underground part. It corresponds to the finding that flavonoid compounds with B-ring tri-hydroxyls do not accumulate in the roots. Existing studies have verified that CsLAR, CsANS, and CsANR can catalyze catechin synthesis[39,40]. CsLARs can catalyze the formation of simple catechins from dihydroflavonols[41]. CsANRs catalyze the synthesis of intermediates, thus promoting the accumulation of proanthocyanidins[28]. The results of this experiment exhibit that CsLARa and CsANSa are highly expressed in the aboveground part and extremely low in the underground part. It contributes to the reasons why simple catechins marginally accumulate in the underground part. Moreover, CsANRa is highly expressed in roots, which explains why proanthocyanidins can be accumulated heavily in roots.
The results of the enzymatic reaction conducted by Zhao et al.[42] disclose that CsSCPL5 is a non-catalytic chaperone protein, and CsSCPL4 collaboratively regulates the galloylation of cis- and trans-flavan-3-ols in tea plants. The expression of CsSCPL is high in shoots and low in other organs. Therefore, ECG and EGCG are not accumulated in the underground part, the same as the heat map of flavanols in the two varieties of tea seedlings.
In addition, the results of PCA analysis revealed that the accumulation patterns of flavonoids in aboveground (shoot and epicotyl) and underground parts (root and hypocotyl) were consistent separately (Fig. 5c). In summary, the aboveground part mainly accumulates B-ring di/tri-hydroxyl catechins, gallate catechins monomers, and polymers, while the underground part concentrates B-ring di-hydroxyl catechins and special flavonol glycosides. Based on these findings, the synthesis pattern of flavonoid biosynthesis in tea seedlings was summarized (Fig. 8).
-
According to quantitative and qualitative detections, the amounts of flavonoids accumulated in the same organ are slightly different among stages and significantly vary with organs. The accumulated amounts of total polyphenols, flavanols, and flavonols in the aboveground part (shoot and epicotyl) are prominently higher than those in the underground part (hypocotyl and root tip). PAs are mainly concentrated in the epicotyl segments. The results of TOF-MS and UPLC-QQQ-MS/MS show that the types of flavonoids accumulated in aboveground and underground parts are diverse. The aboveground part mainly accumulates B-ring di/tri-hydroxyl catechins (EC, C, GC, and EGC), gallate catechins monomers (ECG and EGCG), and polymers, while the underground part amasses B-ring di-hydroxyl catechins (EC and C) and special flavonol glycosides. The qRT-PCR analysis implies that CsF3'5'Ha, CsSCPL4, and CsSCPL5 are hardly expressed in the underground part.
-
The authors confirm contribution to the paper as follows: study conception and design: Jiang X, Li W, Gao L, Wang H, Xia T; experiments performed: Li W, Lai S, Jiang X, Kong D, Wen Y; data analysis: Li W, Jiang X, Wen Y; draft manuscript preparation: Jiang X, Li W, Wen Y, Lai S. All authors reviewed the results and approved the final version of the manuscript.
-
Due to administrative requirements, the original data of the experiments during the research period of the project are not available to the public, but available from the corresponding author or the first author upon reasonable request.
This work was supported by the Natural Science Foundation of China (32372758 and U21A2023), the College Students' Innovative Training Program of Anhui Province (S202110364008), and the Youth Science and Technology Talents Support Program (2020) by Anhui Association for Science and Technology (RCTJ202010). Thanks to Anhui Huangkui Tea Co., Ltd. for providing 'HK' seeds.
-
The authors declare that they have no conflict of interest.
- Copyright: © 2024 by the author(s). Published by Maximum Academic Press, Fayetteville, GA. This article is an open access article distributed under Creative Commons Attribution License (CC BY 4.0), visit https://creativecommons.org/licenses/by/4.0/.
-
About this article
Cite this article
Li W, Wen Y, Lai S, Kong D, Wang H, et al. 2024. Accumulation patterns of flavonoids during multiple development stages of tea seedlings. Beverage Plant Research 4: e013 doi: 10.48130/bpr-0024-0006
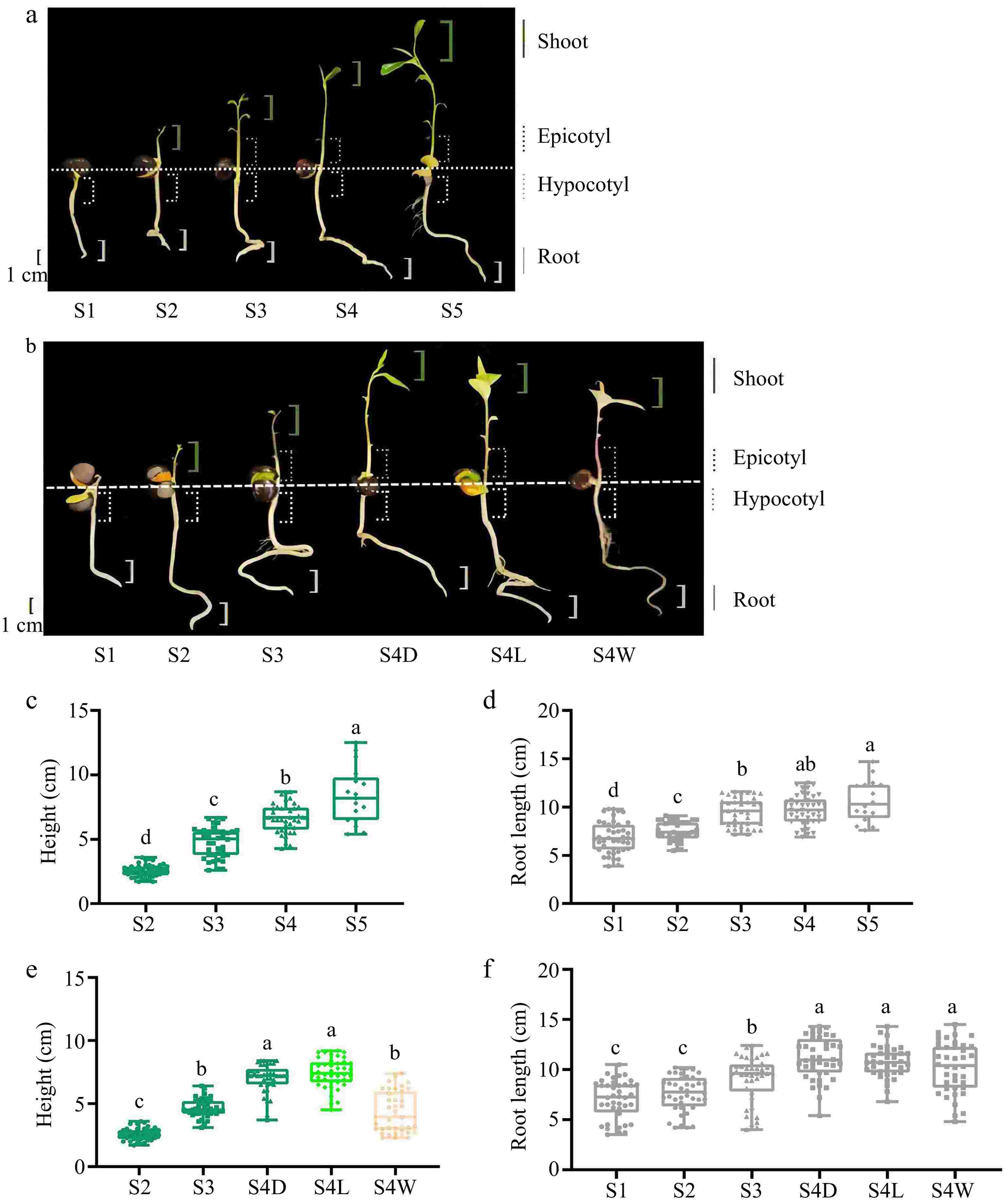