-
Essential oils (EOs) are mostly known as volatile oils or essences, and plant secondary metabolites that are hydrophobic fluids containing volatile aromatic compounds[1]. EOs can be extracted from the aromatic parts of plants such as flowers, leaves, buds, and bark. In plants, EOs act as survival mechanisms against different microbes, fungi, and herbivores. Their aroma also attracts pollinators to help plants reproduce[2]. EOs are a mixture of hydrophobic volatile secondary metabolites from plants with fascinating properties, including distinctive antioxidant and antimicrobial properties. EOs have attracted scientists due to their potential for shelf-life improvement due to their antimicrobial properties and suitability as a green additive to replace synthetic products and their side effects[3]. The distinctive preservative properties of EOs provide solutions to various challenges faced in food preservation. In addition to shelf-life improvement of different food materials, EOs contribute to flavor enhancement in food industry applications. Despite the rich potential of EOs, scientists encounter various issues in handling processes, such as (i) oxidation of EOs due to their sensitivity to light, oxygen, and heat; (ii) the solubility of EOs is low; (iii) the volatile nature of EOs causes limited bioactivity; and (iv) their active binding with other food matrices such as lipids, carbohydrates, and proteins results in high dose requirements. Recently, scientists have been working on improving the biological abilities of EOs by encapsulating them using nanotechnology.
The global demand for natural, sustainable, and synthetic additive-free food products is rising, increasing the pressure on food industry scientists to find green alternatives to synthetic chemical preservatives, natural flavors, and aroma additives. Nanoencapsulation of EOs undeniably offers a promising solution to remarkably enhance stability and bioavailability. Moreover, the meticulously controlled release mechanism unequivocally makes the consumption of encapsulated EOs significantly more effective in the food preservation process. As a result, nanoencapsulation boldly addresses the challenges encountered in food preservation and shelf life extension, while minimizing the need for synthetic chemical additives in food products to naturally maintain quality. Consequently, meeting consumer demands for natural and safe food products. The introduction of nanoencapsulation into the food industry undeniably holds extraordinary potential for pioneering methods of food preservation and safety, thereby unequivocally contributing to sustainable global food practices.
Bioactive compounds from plants can be stabilized and enhanced using nanoencapsulation. This process involves enfolding EO components into nanometer-sized carriers for protection and enhanced finality. It can also increase the physical stability of active ingredients and protect them from interfacing with other food ingredients[4].
Nanoencapsulation of EOs and their bioactive compounds enhances stability, activity, and lower volatilization, eventually improving their biological potential and providing solutions to challenges faced in the consumption of EOs in the food industry[5]. Figure 1 summerzie problems faced in applications of EOs in the food industry and the mechamism of actions of EOs if used properly. Nanoencapsulation can be adopted through several nanostructure systems, such as nanoparticles or nanoemulsions, which are up-and-coming[6]. Nanocapsules increase the passive cellular absorption mechanism, leading to a reduction of mass transfer resistance and an increase in its antimicrobial activity compared to microcapsules. Some advantages of nanoencapsulation include a large surface-volume area, behavior and precise release kinetics of EOs, mass transfer, and bioactive constituents to the targeted food system[7]. The process of nanoencapsulation includes the bioactive compounds to be coated within a matrix, which can be natural or synthetic[4]. The chosen matrix then isolates targeted ingredients from exposure to the external environment[8]. Several nanoencapsulation techniques, such as spray drying, solid lipid nanoparticles, and coacervation, are employed to counteract limitations and enhance the biological activity of EOs[9]. In the food industry, encapsulated EOs are directly applied as antimicrobial agents and active packaging to improve the shelf life of packaged food[10].
Figure 1.
Essential oils mechanism of action and the challenges faced in food industry application for their consumption.
Xavier et al.[11] encapsulated EOs from Cinnamodendron dinisii that enhanced the preservation of beef. Silva et al.[12] encapsulated EOs from clove oil to treat vaginal candidiasis; the nanoemulsions were prepared for enhanced colloidal stability with suitable pH for the treatment. EOs from oregano were encapsulated by Corrado et al.[13] using the solvent evaporation technique, which had higher biological activity against Micrococcus luteus.
Several studies on vital herbs and their component EOs have been carried out in food, biotechnology, and medical fields. Esmaeili et al.[14] incorporated nanoencapsulation of garlic EOs to enhance the shelf life of vacuum-packed sausages, Amiri et al.[15] studied the effects of nano-encapsulated cumin EOs as an antibiotic to promote growth in broiler diets, Hadidi et al.[16] used EOs from Hemp to nano encapsulate in alfalfa proteins to prepare different healthy bioactive agents.
Nanoencapsulation is one of the most versatile methods of the 21st century in the biotechnology and food industry. This review covers the progress over the past few years in the food industry involving nano-encapsulated EOs, mechanisms, and the future expectations from this technology in both sectors. Nanoencapsulation in EOs can be done using several carriers, such as polymer-based nanocapsules, liposomes, nanoemulsions, solid nanocarriers, and molecular complexes.
Numerous review papers have been published in the area of nanoencapsulation of EOs such as Weisany et al.[17] focused on target delivery and the controlled release mechanism of nano-encapsulated EOs. Whereas Sharma et al.[18] focused on one kind of food industry application i.e., food packaging, Chaudhari et al.[19] addressed myotoxin elimination using nano-encapsualted EOs. Lammari et al.[20] discussed nanoprecipitation and its uses in the encapsulation of EOs. This review presents recent advancements in the nanoencapsulation of EOs and their applications within the food industry. It emphasizes using nanoencapsulated EOs as green preservatives, highlighting their enhanced antifungal, antimicrobial, and antioxidant properties. It delves deeply into the mechanisms of action of these nanoencapsulated EOs in food preservation.
This review also thoroughly examines various nanoencapsulation approaches, providing a detailed summary of how traditional methods have evolved and how novel techniques offer superior efficacy. Additionally, it includes a critical assessment of safety, future potential, and challenges associated with using nanoencapsulated EOs, offering readers a forward-looking perspective on the field. Moreover, it presents a single, detailed resource that combines all these aspects, providing the most up-to-date data and discussing innovative modifications in nanoencapsulation methods and their applications in the food industry.
-
Nanoencapsulation is the process in which nanosized particles called nanocapsules are used to encapsulate active substances such as EOs to protect them from environmental degradation, allowing them to be released in a controlled manner triggered by specific conditions for longer periods and enhanced solubility with various solvents.
Approaches for nanoencapsulation of EOs
-
Nanoencapsulation technologies have been classified into two mechanisms to elaborate the nanomaterials: top-down and bottom-up. The top-down technique refers to decreasing particle size using appropriate tools, whereas particle size increases in the bottom-up technique. The bottom-up method includes techniques such as supercritical fluids, coacervation, inclusion complexation, and nanoprecipitation, while the emulsion method is considered a top-down method (Fig. 2). Nanoencapsulation systems can be divided into liquid-liquid, solid-solid, and solid-liquid systems. Different delivery systems were developed to encapsulate bioactive compounds. These nanoparticle delivery systems are specified according to the key factors affecting bioavailability that characterize the contained ingredient. The central delivery systems and their types are discussed in this study.
Techniques such as coacervation, emulsification-solvent evaporation, supercritical fluid, emulsification, nanoprecipitation, and inclusion complexation mainly produce nano-sized capsules with variable sizes (100−1,000 nm) (Fig. 2).
Top-down techniques
-
Emulsion stands out as a highly effective top-down technique for nanoencapsulation, adept at significantly reducing particle size through the application of high shear forces and ultrasound. This method disperses EOs as minuscule droplets within a continuous phase, stabilized by surfactants, ultimately yielding impressive nanoemulsions. Nanoemulsions play a crucial role in enhancing the stability and bioavailability of EOs. However, their practical application is hindered by the coalescence of droplets over time. Therefore, a comprehensive assessment of emulsifier selection is necessary to mitigate any potential impact on the sensory attributes of the final food product.
Bottom-up techniques
-
In bottom-up techniques, nanoparticles are assembled from molecular components. Various methods included in bottom-up techniques are discussed as follows.
Supercritical fluids
-
The current methodology eliminates the need for toxic solvents and utilizes carbon dioxide as a solvent for the formation of nanoparticles. Recent enhancements have significantly improved the stability and economic viability of its application on an industrial scale.
Coacervation
-
This technique forms a coating around the EO droplets by separating the polymer-rich phase from the polymer-poor phase, and is used specifically to study controlled release properties. Recent developments in this method involves the formation of coacervates which are more stable to environmental factors such as pH and temperature.
Inclusion complexation
-
This method primarily involves a host-guest interaction to encapsulate EOs. The environmentally friendly host, cyclodextrin, is utilized to encapsulate through the formation of inclusion complexes, effectively protecting EOs from degradation by environmental factors. Notable advancements in this methodology include enhancing release kinetics in food systems.
Nanoprecipitation
-
This method is widely recognized for its straightforward technique of precipitation, achieved through the rapid mixing of a solution with EOs using a non-solvent, resulting in the formation of nanoparticles with a narrow size distribution. Recently, researchers have dedicated their efforts to enhancing the ratios of solvent to non-solvent and optimizing the mixing conditions to improve yield and facilitate industrial applications.
Comparison and progress of traditional methods
-
Selecting a nanoencapsulation technique is a critical decision, as each method presents distinct advantages and limitations in its applicability to the food industry. Industrial applications necessitate simpler and more efficient methods, such as emulsification, to facilitate production at higher volumes and reduced costs. It is important to note, however, that the use of surfactants in this process may potentially impact the sensory properties of the food product, as they are essential for stabilizing the emulsions. Conversely, bottom-up techniques offer advantages in controlling particle size and morphology and achieving superior encapsulation of EOs, thus safeguarding them from environmental degradation. Nevertheless, these methods are not cost-effective, and their complexity presents challenges when scaling up for industrial applications.
The utilization of supercritical fluids represents a significant advancement embraced by scientists to enhance the scalability of complex bottom-up techniques. This has led to improved efficiency and environmental impact. The integration of hybrid approaches represents a significant advancement in this field, combining both top-down and bottom-up techniques. Recent studies have focused on emulsion formation followed by nanoprecipitation to create more stable nanoemulsions with high encapsulation efficiency. Furthermore, recent research into the use of modified cyclodextrins as encapsulating agents to enhance functionality and ensure product safety. In conclusion, traditional methods that are commonly employed are gradually transitioning towards optimization to achieve improved and safer outcomes with enhanced efficiency for applications in the food industry. Future research endeavors should prioritize developing modified methods and novel materials to address existing limitations.
Active packaging is a modified method that involves the incorporation of active agents into packaging film to improve the shelf life of food products. This approach encompasses advanced protective properties, including antioxidants and antimicrobial effects. It obviates the use of chemical additives that may migrate from packaging to food items and minimizes excessive industrial processes to safeguard food items. The strategy comprises the implementation of ethylene absorbers, carbon dioxide emitters, antioxidant packaging, antimicrobial packaging, moisture absorbers, and O2 absorbers[18]. This approach encompasses top-down techniques involving the incorporation of nano-encapsulated EOs into the polymer matrix of packaging film, as well as bottom-up techniques that typically entail the molecular assembly of nanoparticles or encapsulated structures directly within the film material. Sharma et al.[18] discussed nano-encapsulated EO incorporation in active packaging film to target food industry applications in detail.
Emulsion, supercritical fluid technique, and coacervation are mostly used for lipophilic and hydrophilic compounds. Some methods, such as complexation, nanoprecipitation, and emulsification-solvent evaporation, are used primarily to encapsulate lipophilic compounds. Nanocarriers based on different materials are divided into three main categories to encapsulate EOs according to their applications in the food industry (Fig. 3).
Lipid-based nanoencapsulation
-
Lipid-based nanoencapsulation is one of the most advantageous and rapidly developing systems for nanoencapsulation. Lipids, as the natural ingredient, are used as the oil phase in different formulations, either external or internal. One of the main benefits of this technique is that it is prepared from natural ingredients and can entrap materials with different solubilities. Also, it can protect ingredients from oxidation caused by free radicals, pH changes, and enzymes. Lipid-based nanocarriers can accommodate both water-based and lipid-based materials. These nanocarriers can be directed inside the body by active and passive mechanisms based on size. Lipid-based nanoencapsulation systems include liposomes, solid-liquid nanoparticles, nanostructured lipid carriers, and emulsions. EOs are mainly encapsulated using lipid-based encapsulation systems to enhance their stability and biological activities by controlling release and targeting effects.
Nano-liposomes, vesicles, and emulsions
-
Liposomes are the most studied nanoencapsulation system, and they are studied for several purposes. Liposomes comprise spherical vesicular self-assembled, consisting of one or many concentric phospholipidic bilayers containing an internal aqueous layer. They can be divided into: (i) one bilayer of unilamellar vesicles (ULV), (ii) multilayer vesicles containing non-concentric bilayers MVV, and (iii) multilamellar vesicles containing several bilayers (MLV)[14]. Liposomes consist of variable sizes ranging from 20 nm to up to 1 μm[21]. They have lipophilic palisades and hydrophilic compartments to accommodate hydrophilic and lipophilic compounds. Liposomes are the best carrier for EO components because they enhance solubility and stability. Recently, different scholars have used the RESS method. The conventional rapid expansion of the supercritical solution (RESS) method involves the precipitation of solutes as microplates by dissolving them in supercritical solvent and then expanding the solution into the atmosphere. The modified RESS method involves dissolution before processing[21]. A thin film hydration method combined with sonication has been used by Esmaeili et al.[14] on garlic EO to enhance microbial growth inhibition with nano-sized 101 and 250 nm liposomes, respectively. Ben-Fadhel et al.[21] and Chavoshi et al.[22] prepared nanoliposomes of 132.4 and 167.8 nm by homogenization from chrysanthemum EO (CHEO) and Odecumbens EO to enhance the antibacterial activity of C. jejuni. Thyme EOs has been encapsulated by Al-Moghazy et al.[23] to improve the shelf life of cheese. Cinnamon oil and citrus extract properties were enhanced to prepare natural preservatives by Ben-Fadhel et al.[21] by formulating nanoliposomes. Niosomes from Lippia citriodora EOs has improved food preservation.
Liposomes, with their lipid bilayer structure, create a suitable carrier for EO encapsulation and provide a distinctive prospect to enhance biological activities, i.e., antimicrobial, antifungal, and antioxidant for food preservation and shelf-life enhancement. The ability to encapsulate hydrophilic and hydrophobic compounds makes them superior to others in the food industry. Targeting food preservation liposomes can offer protection of sensitive components, stability, and the controlled release of EOs resulting in enhanced biological activities to provide improved shelf life of food products with great quality and nutritional value.
Amphiphilic molecules are used to form vesicles; they are represented by natural or synthetic phospholipids (liposomes) or nonionic surfactants (niosomes)[24]. Vesicles can be of various sizes, ranging from 50 nm to several macrons with spherical shapes[24]. Pharmacokinetics and biodistribution can be affected by the size of vesicles. The main compound in fluidizing by layer is cholesterol, which is not used to form a bilayer. Vesicles possess high biocompatibility, biodegradability, and versatility because they can carry hydrophobic and hydrophilic EOs and drugs, further enhancing stability, solubility, controlled release, and less toxicity[25]. Vesicles possess ease in surface modification to get passive or active target delivery of EOs with prolonged and lifetime circulation, whereas the main limiting factor is the leakage of active ingredients due to the release from the vesicles and the low colloid stability. Palmas et al.[26] prepared nanovesicles using sonication to target Citrus limon var. pompia essential oil to inhibit the proliferation of the cariogenic bacterium. EOs from Rosemary (Rosmarinus officinalis L.), thyme (Thymus vulgaris L.), savory (Satureja montana L.), three species of oregano (Origanum virens, O. majoricum Camb., and O. vulgare L.) were encapsulated in niosomes to control Aspergillus flavus growth by García-Díaz et al.[25] for food preservation purposes.
Nanovesicles offer high encapsulation efficiency to EO components, resulting in carrying greater amounts of EOs to the targeting sites while protecting them against degradation from light oxygen and other environmental factors, which eventually can provide a significant change in the biological activities of the encapsulated compound for food preservation purposes. Moreover, nanovesicles provide a unique quality of carrying multiple bioactive compounds with synergistic effects, which can be notable for enhancing multiple biological activities to address various food preservation challenges in the future.
Nanoemulsions can be prepared by mixing two immiscible liquids with the help of emulsifiers to make them stable with a size of < 100 nm[27]. Due to their small size, nanoemulsions possess higher kinetic stability even for more extended periods of years than microemulsions[28]. Formation of nanoemulsion includes high-energy methods (such as ultrasonication, high-pressure homogenization, and micro fluidization) and low-energy methods (solvent dispersal); both approaches can be employed depending upon the materials and active ingredient stability[29]. Davila-Rodriguez et al.[30] used high-frequency ultrasound and homogenization to encapsulate cinnamon EOs to enhance antimicrobial, antifungal, and antioxidant activities.
Formation of nanoemulsion can be opted in (i) oil in water in which water is the external aqueous phase mixed with oil droplets with emulsifier or stabilizer; (ii) multiple emulsions prepared in different manners: oil-water-oil and water-oil-water; (iii) multilayered emulsions in which polyelectrolytes surround nanometric oil droplets.
The stability and bioactivity of hydrophobic EOs can be increased by encapsulation of O/W emulsions. EOs from thyme have been encapsulated in nanoemulsions for several purposes, such as improved food preservation and enhanced antimicrobial antifungal and antioxidant effects[29]. The antibacterial activity of various lime EOs was enhanced by nanoemulsion encapsulation by stirring[31].
The micro fluidization technique was adopted by Salvia-Trujillo et al.[32] and Shahbazi[33] to encapsulate clove oil EOs into nanoemulsion to achieve improved biological activities.
Encapsulating EOs in emulsions provides effective dispersion to target active sites for better preservation results. Biological activities of EOs can be enhanced by this effective dispersion, i.e., contact between EOs and food matrices. Emulsion offers enhanced stability and bioavailability by increasing the solubility of EOs to achieve high absorption and, thereby, increasing the biological activity of carried components. The versatility in formation and application is another important aspect of emulsions that allows various EOs incorporation with unique properties. Emulsions are tailorable according to their food preservation applications by optimizing the biological activity of EOs. In addition, their cost effectiveness has led them to be used industrially worldwide in the food industry.
Solid-lipid nanoparticles (SLNs) and nano-structured lipid carriers (NLCs)
-
NLCs and SLNs are composed of nanosized particles using solid lipids at room temperature. The lipids in this nanocarrier system may consist of a broad range of lipid or lipid-like molecules like waxes or triacylglycerols with a diameter size of 50 nm and 1 μm. The ingredients to be carried can be solubilized in the core's outer part, making it immobile to protect it from leaking and sustained release, giving active ingredient stability against degradation[34]. Hot and cold homogenization prepares liquid nanoparticles. Homogenization can be done using a high-pressure homogenizer, ultrasonic probe/bath/jet, or microfluidizer.
Recently used techniques such as sonication and high-shear homogenization can be cost-effective. Still, the unstable dispersion renders and low encapsulation efficacy make these techniques used in limitations for EOs encapsulation[35]. Furthermore, high-pressure homogenization is the most used method to produce SLN and NLCs; nonetheless, it may result in various particle sizes[36]. Several studies have been done to prepare SLNs for EO encapsulation. Recently, researchers have been using a modified method that couples ultrasound with high-shear homogenization. Safaeian Laein et al.[37] successfully enhanced the antifungal and antibacterial activities of Peppermint EOs and Eugenia caryophyllata EO into solid lipid nanoparticles (SLN) using a sonication bath attached to the high-shear homogenization method.
Hot high-pressure homogenization can cause heat degradation of EOs and alter their properties; hereafter, cold, high-pressure homogenization is one of the most suitable techniques that can be adopted under optimized conditions to sustain the quality of EOs[34]. Alpha-pinene, citral, geraniol, or limonene encapsulated in SLNs with size from 89.73 ± 0.312 nm to 140.767 ± 1.620 nm under controlled conditions for the drug delivery[38]. SLNs can increase bioavailability by reducing the toxicity of different organic solvents. They are cost-effective and can be produced at the industrial level. SLNs can be divided into three classes: (i) The homogenous matrix model is the matrix in which the core carries active ingredients; (ii) An active ingredient enriched model is one in which the outer shell contains active ingredients with lipids, and the inner core is free from drug/active ingredients; (iii) The active ingredient enriched model is the matrix in which the active ingredient is saturated closely to its limit in lipid, resulting in precipitation in the core, and the lipid covering is molded[39].
NLCs are similar colloidal systems to SLNs, with only a difference in the formation of solid and liquid units, resulting in the forming of a shambolic matrix enclosed in surfactants[40]. Hot melt homogenization prepared NLCs from Carum carvi and lavender oil for improved anti-inflammatory properties[40]. This system avoids the premature release of active ingredients and has high encapsulation efficiency. Antibacterial and antifungal activities of EOs from Lippia sidoides, cinnamon and Rosmarinus officinalis, Lavandula intermedia, and Sumian Origanum vulgare were enhanced by encapsulating them into NLCs by high-pressure homogenization method. Sonication attached to high-pressure homogenization was employed to make NLCs in many recent studies[36].
SLNs and NLCs have solid lipid matrices with high loading capacity, which can lead to encapsulating larger amounts of active ingredients, resulting in the controlled release delivery of a significant amount of preserved EO components to target sites, which enhances the biological activity in food preservation. The biocompatibility of SLNs and NLCs makes them safe and suitable for food applications through effective interaction with biological systems. Moreover, these carriers have an especially versatile formulation that enables them to carry various compounds with different properties with tailored delivery systems that can target optimization of specific biological activity of the encapsulated EOs for food preservation application.
Polymer-based nanoencapsulation
Dendrimers, nanofibers, and micelles
-
Dendrimers are spherical nanoparticles of variable sizes ranging from 2−200 nm in diameter. They are three-dimensional spheres of a core with successive series of branches recognized as first-generation and second-generation. Dendrimers comprise three parts: core, interior, and end groups. The main characteristic of dendrimers is that the active ingredients can be incorporated into both branch surfaces and inside the core by covalent or electrostatic interaction. Apart from other advantages of nanoencapsulation, it has a unique advantage in that it is a monodisperse that offers repeatable pharmacokinetic characteristics. Basic dendrimers have limitations such as ineffective release of active compounds, unstable loading of hydrophobic molecules, and high cost of production. Recently, new categories have been formulated to address these issues. EOs can be chemically attached to chemical moieties or encapsulated in internal cavities. Different derivatives of amine, such as poly (amidoamine) and poly (propylene imine), are the basic units for preparing dendrimers. However, they are used to make their surface functionalized poly-L-glutamic acid and polyethylene glycol. Third-generation dendrimers were prepared to enhance the stability of drug delivery antibacterial activity of tea tree oil, thyme, and cinnamon EOs[41]. Stirring and electron spinning are recent methods to explore food preservation, storage, and packaging with Citronella EOs, Blume Cymbopogon winterianus and Jowitt, and Eucalyptus EO[42]. A spontaneous complexation method encapsulated cinnamon and citronella EOs in third-generation polyamidoamine (G3 PAMAM) dendrimer[41]. Stirring coupled with the electrospinning process is a recently used method by researchers to encapsulate tea tree and oregano EOs, which leads to enhanced antibacterial and antifungal activity in food preservation applications[43]. The nature of functional groups and dendrimer generation can possess a certain type of cytotoxicity[43]. Other unique properties include water solubility, inner core availability, and high branching, making them capable agents for EO delivery applications. Divergent and convergent are the two main methods employed to synthesize dendrimers. Under optimized conditions, dendrimer synthesis depends on two basic reactions: (i) Williamson ether synthesis and, (ii) the Micheal reaction[44]. Many novel techniques are also used, such as organosilicon chemistry, organo–phosphorous chemistry, or solid phase synthesis[44]. The covalent bond formation is a basic similarity between the two dendrimer-making methods. Hydrophobic building blocks of dendrimers are (poly) propylene glycol, (poly) aspartic acid, (poly) β-benzyl-l-aspartate, (poly) lactic acid, (poly) ε-caprolactone, and (poly)trimethylene carbonate whereas, hydrophilic building blocks include polyglycerol, (poly)2-oxazoline, (poly)ethylene oxide), (poly)methacrylic acid, amino acids, carbohydrates (poly)glycerol, (poly)2-oxazoline, (poly)ethylene oxide, (poly)methacrylic acid, amino acids, and carbohydrates[45].
Dendrimers have specific alterable structures to encapsulate EOs with efficient encapsulation efficacy due to multivalent structure and interior void space to carry larger amounts of EOs. Dendrimers offer various unique benefits to enhance the food preservation ability of encapsulated compounds which include an alterable controlled structure with controlled release of encapsulated compounds and high loading capacity to enhance the stability and targeted delivery of EOs.
Polymeric fibers have a small pore size and a larger surface area within the size range of 10−100 nm[46]. Electrospinning is the most common and promising technology to encapsulate EOs into biocompatible polymeric nanofibers to enhance stability and biological activities[46]. Oregano, thyme, Ziziphora clinopodioides , rosemary, and green tea extract are encapsulated in nanofibers to enhance their targeted biological activities, such as antimicrobial films in food industry packaging. Coaxial ES is another optimized method used for nanoencapsulation of EOs into nanofibers with great antimicrobial ability and hence used in packaging in the food industry[47,48]. The antifungal activity of cabreuva, clove, and thyme EOs by encapsulating extracts in nanofiber mats using electron spinning. Natural polymers are preferred over synthetic or other materials to reduce environmental, health, and food-borne problems. Karami et al.[47] explored stirring with nanofibers can reduce the aroma of EOs, make them stable, and protect them from oxidation degradation[49]. Pure EOs incorporated into nanofibers enhance their ability to control pests and lower toxicity towards other organisms[50].
Nanofibers have unique properties such as a high surface area to volume ratio to adsorb and interact with EOs for high encapsulation efficacy and precise delivery, excellent mechanical properties to preserve the encapsulated compounds during storage and processing, changeable pore size and porosity to optimize controlled release ensuring EOs to deliver on specific target sites for enhanced preservation abilities. Nanofibers offer versatile and biocompatible formulations for food applications, which can lead to carrying various active compounds with suitable food applications to tackle the challenges of food preservation.
Micelles can be used as passive targeting by encapsulating hydrophobic bioactive compounds in the nucleus[51]. Core-shell structures can be formed when amphiphilic polymeric molecules bind to an aqueous medium. These nano vectors can range from 20−200 nm. Micelles formation can occur naturally when the concentration of monomers is up to a critical micellar concentration. Ganguly et al.[52] enhanced the antimicrobial effect of tea tree EOs by preparing an EO and NaCl mixture. Luo et al.[51] prepared micelles to explore advantages in the food industry by enhancing cinnamon EO food safety and antimicrobial activity (CEO). Generally, mixing is a commonly adopted method by researchers[51]. Ultrasonication has also been carried out by Soliman et al.[53] to study geraniol for its enhanced anti-inflammatory activity. Polymeric micelles have many unique properties such as stability, love cost nano vectors, safety, and the capability of being used for all the administration routes[51].
Micelles have various benefits in enhancing the biological activities of EOs with enhanced stability and solubility to solubilize hydrophobic EO components, resulting in effective dispersion in food matrices. Micelles offer high bioavailability of encapsulated compounds by enabling the adsorption and transport across biological barriers due to their small size, leading to high biological activity of encapsulated compounds. Micelles also offer versatile formulations to carry various EOs and other compounds for their targeted applications. This can allow the preparation of an optimized delivery system to get the enhanced biological activity to target food preservation purposes industrially.
Molecular complexes
-
The complex structure is formed by the physical association between the host and the active ingredient molecules, resulting in improved stability and solubility[54]. Molecular complexes can be formed to encapsulate EOs by using cyclodextrins as nanocarrier materials[55]. CDs' most unique feature is that they can make water-soluble inclusion complexes with the most hydrophobic molecules[55]. Herbs such as thyme[55], oregano[54], and gangal root extract[55] were recently studied for their enhanced antimicrobial activities. Whereas tea tree oil[56], labdanum EO (Cistus ladaniferus)[57] and cinnamon EOs (CEO)[58] were studied for their improved stability and solubility properties in food preservation. A few studies have been done for food industry applications, specifically for the preservation and storage of food, considering the enhancement of the antifungal activity of orange and cinnamon EOs[58,59].
Assessing the complexation ability can ensure the efficiency and viability of the process[58]. CD complexations are widely used in the food, cosmetics, and pharmaceutical industries, and their significant advantages are: (i) protection against oxidation in both light and heat decomposition, (ii) avoidance of losses from sublimation and evaporation, (iii) removal of undesired tastes and odors; and (iv) helping to protect active ingredient interaction with additives.
Molecular complexes, particularly cyclodextrin inclusion complexes, have several key benefits in target food preservation by enhancing the biological activities of encapsulated EOs components. Like other nanocarriers, they enhance the solubility and stability of EOs for better protection from degradation by heat, light, and oxygen, with enhanced water solubility to increase high distribution in aqueous food systems. Controlled release from these complexes can lead to prolonged antimicrobial and antioxidant effects by avoiding the volatility and instability of the compound. The high bioavailability of these carriers offers a higher proportion of active ingredient delivery to targetted sites, resulting in enhanced biological activities of EOs. The synergistic effects of combining EOs with other bioactive compounds further enhance their food preservation applications in the form of edible coatings and thin films to provide protective barriers.
-
The process of nanoencapsulation of EOs presents numerous advantages for the food industry, including preservation, natural flavor enhancement, and improved stability. This cutting-edge technology serves to safeguard EOs from environmental degradation, thereby augmenting their inherent properties, such as antimicrobial activity, aroma, bioavailability, stability, and solubility. The subsequent sections delineate the specific benefits of nanoencapsulation, emphasizing its impact on solubility, stability, targeted delivery, and overall effectiveness in food preservation applications.
Controlled release of nano encapsulated EOs
-
Nanoencapsulation can help control the release of EO in a controlled manner for significant effects. Several mechanisms are discussed by which the encapsulated compound is released to the target, such as; diffusion, erosion, fragmentation, and shrinking/swelling[60]. Diffusing of EOs from the matrix to the surroundings is the diffusion mechanism, whereas the erosion mechanism involves erosion of the outer part of the carrier to expose the EO with the help of chemical hydrolysis or enzymes[61]. Fragmentation is the cutting of carriers into fragments by mechanical forces. Swelling includes the increase in the pore size of the carrier and the release of encapsulated compound in the surroundings[60]. These processes can be initiated by different factors such as heat, moisture, pH, light, or temperature, which leads to damage to the carrier for delivery. Rajkumar et al.[62] studied the controlled release of peppermint EOs using pH 3−7 and concluded nanocapsules were degraded slowly in two stages. The initial stage had released up to 47.4%, and the second stage led to the release of EOs up to 62.4% over 72 h to target pest control in grains. Soltanzadeh et al.[63] prepared chitosan nanoparticles encapsulating lemon grass EOs and studied in-vitro-controlled release using high-temperature. Results explained that encapsulation results in high-temperature stability of EOs up to 252 °C compared to pure LGEOs at 126 °C. Min et al.[46] successfully explored humidity-dependent control release of thyme EO for antimicrobial packaging fils for shelf life enhancement of strawberries. Controlled release systems play a crucial role in the food industry, ensuring a consistent release of antimicrobial drugs to preserve food and extend its shelf life, thus contributing to enhanced food security. Similarly, in the pharmaceutical sector, controlled release strategies optimize the delivery of medicinal substances, leading to improved patient adherence and a reduction in the frequency of medication administration.
Enhanced stability
-
The encapsulation of EOs serves to shield them from natural degradation caused by environmental factors like heat, light, oxygen, and moisture. Nanoencapsulation establishes a protective barrier between the volatile molecules and the surrounding environment, thereby assisting EOs in maintaining their chemical integrity and potency over time. Numerous studies have underscored the benefits of the enhanced stability of nanoencapsulated EOs[6]. For instance, a study compared the antioxidant prowess of nanoencapsulated orange peel EO in soya bean oil during storage, revealing that the nanoencapsulated OPEO exhibited a 39.8% higher antioxidant capacity compared to the non-encapsulated oil, which demonstrated a 24.6% capacity[64]. These findings substantiate the notion that nanoencapsulation can endow EOs with enhanced antioxidant properties and fortify them against oxidative damage, culminating in an extended shelf life.
Improved solubility
-
EOs, due to their hydrophobic nature, present a challenge as they are not soluble in water or many solvents, constraining their use in aqueous formulations. However, encapsulating EOs in nanocarriers offers a promising solution to enhance their solubility and revolutionize their integration into the food industry. In applications such as beverages and creams, the improved solubility of EOs can greatly amplify the quality of water-based products, ensuring consistent dispersion and maximizing their benefits. Reducing particle size to the nanometer scale can significantly improve the uniform distribution of hydrophobic particles. Coating oil droplets with hydrophilic materials stabilizes them in an aqueous environment by preventing aggregation. Furthermore, the process of nanoencapsulation can effectively modify the chemical environment, enhancing solubility and allowing for controlled release. These advancements open up new avenues for their application within the food industry.
Targeted delivery
-
Nanoencapsulation is a promising approach for delivering EOs to targeted sites through controlled release mechanisms. These sites may exist within the human body or may be specific locations within the environment. The advantages of nanoencapsulation are particularly significant in the medical industry, and its implementation within the food industry has the potential to transform preservation techniques. In agriculture, targeted delivery can facilitate the direct application of pesticides or insect repellents to specific pests, thereby minimizing the impact on beneficial organisms and the environment.
-
Nanoencapsulation is revolutionizing the food industry by providing new ways to use EOs. EOs have traditionally been difficult to use due to their sensitivity, but nanoencapsulation protects them and makes them soluble in various solvents, making them ideal for beverage use. This technique offers a range of applications, addressing specific challenges in food preservation, and has the potential to transform the industry.
Nano-encapsulated EOs as green food preservatives
-
Nanoencapsulation of EOs has opened new doors to novel applications of EOs in the food industry, such as enhanced insecticidal and larvicidal properties, which have made them strong candidates for eco-friendly and greener preservatives for food materials[65]. Polymer-based nanoencapsulation of EOs and their components has shown greater efficacy[66]. Food and agricultural industries are focusing more on preparing new formulations of stabilized nano-encapsulated EOs using lower doses to resist insects and fungi. Emamjomeh et al.[67] studied enhanced contact toxicity of Eucalyptus globulus Labill and Zataria multiflora Boiss EOs against the pest infestation of Ephestia larvae. Polymeric nanoparticles loaded with Mentha spicata EOs were employed to enhance insecticidal activity in various food sectors. Valencia orange leaf EOs showed enhanced insecticidal activity in nano-hexosomal formulations[3]. Chitosan and gum Arabic nanoencapsulation of Rosemary EOs showed long-term pesticidal effects for stored food materials[68]. Lemon peels and cinnamon oil EOs nanoformulations were recently used as alternative bioinsecticides to control Agrotis ipsilon and rice moths[69]. Hosseini et al.[70] prepared chitosan nanogels from Shirazi thyme EOs to apply as bio preservatives in Iranian white cheese. Das et al.[71] explored Carum carvi EOs emulsions as an eco-friendly alternative to store herbal raw materials from fungus attack. Meat safety and preservation was employed by Gorzin et al.[65] using EOs from basil. Rice storage quality was enhanced by preparing chitosan nano biofilms from Apium graveolens EOs[72]. Fungal attack on stored millet was addressed by Singh et al.[66] using chitosan-based Ocimum americanum EOs as green preservatives. Low-fat foods such as yogurt drinks and nanoencapsulation of Nepeta crispa EOs were used as natural preservatives[73].
Antimicrobial and antifungal activities
-
Food storage and safety include insecticidal, antifungal, and antimicrobial agents. EOs exhibit great fungitoxicity and mycotoxin inhibition efficacy compared to synthetic chemical products. In the past, direct application of EOs was made as food antibacterial agents, but it faced many challenges, such as degradation, insolubility in water, and effects of EO aroma on the quality of food products[74]. Table 1 reports the latest nanocarriers and their antimicrobial actions against various food-borne pathogens.
Table 1. Different EOs are encapsulated in various nanocarriers to enhance their biological activities for various food industry applications.
Sample Carrier Biological activities Ref. Basil EOs Nanoliposomes Antimicrobial [86] Clove (Syzygium aromaticum) oil, black seed (Nigella sativa) oil, thyme (Thymus vulgaris) oil, garlic (Allium sativum) oil, rosemary (Rosmarinus officinalis) oil and green tea (Camellia sinensis) Nanoliposomes Antimicrobial pathogenic E. coli [87] Eugenol isoeugenol estragole and trans-anethole Cyclodextrin and liposomes Antibacterial activity against E. coli, Staphylococcus epidermidis [88] Alpinia galanga EOs Liposomes (EO-Lip) Antioxidant and bacteriostatic properties [89] Achillea millefolium EOs (A. millefolium EOs) Nano-liposomes/
nano-niosomesAnitmicrobial [90] Litsea cubeba EOs (LCEO) Inclusion complex (IC) Antifungal activity against P. italicum and
G. citri-aurantii[91] Cinnamon oil Inclusion complex
(IC beta-cyclodextrinAntifungal activity against Fusarium oxysporum f. sp. cubense-TR4 (Foc-TR4) [92] Tea tree oil (TTO) Inclusion complexes Antifungal activity against B. cinerea Eucalyptus globulus and lemongrass
(Cymbopogon flexuosus) EOsNanoemulsions Antifungal activity against Candida albicans,
Cymbopogon flexuosus[93] Croton caracara 7-hydroxycalamenene-rich EOs (NECC) Nanoemulsions Antifungal activity against Candida albicans, Mucor ramosissimu [94] Essential oils of eucalyptus, clove, thymus Nanoemulsions Antifungal activity against Alternaria alternata [95] Green tea essential oil (GTO) Nanoemulsion Antifungal activity against Magnaporthe oryzae [96] Cinnamaldehyde Cinnamaldehyde-loaded liposomes Antibacterial activity against Staphylococcus aureus [97] Citrus limon var. pompia essential oil Phospholipid vesicles. Antimicrobial and antifungal activity against Escherichia coli, Pseudomonas aeruginosa, Staphylococcus aureus, and Candida albicans [98] Mentha pulegium essential oil (MPO) Nanostructured lipid carriers (MPO-NLCs) Antiinflammatory, antibacterial activities against Escherichia coli and Pseudomonas aeruginosa [99] Sunflower, olive, corn, peanut, coconut, castor,
and sweet almond, and essential (eucalyptus) oilsNanostructured lipid carriers (NLC) Antimicrobial and anti-inflammatory activities [100] Peppermint EO Solid lipid nanoparticle Antibacterial activity against food-borne bacteria Listeria monocytogenes, and Escherichia coli O157:H7 [101] Cinnamon oil Solid lipid nanoparticles (SLN) and chitosan nanoparticles Antibacterial actions against Klebsiella pneumonia and Escherichia coli [102] Oliveria decumbens Vent. (OEO) and Basil EOs Nanocapsules Enhanced antimicrobial and antioxidant activity on minced beef preservation [65] Clove (Eugenia caryophyllata) EO NLCs Films containing essential oils for the preservation of apples, strawberries, and ground beef [103] Apium graveolens EOs Nanobiopolymer Antifungal activity for rice. Food safety application [72] Limonene Solid lipid nanoparticle (SLN) Controlled release, antioxidant activity [104] Thyme oil (TEO) Polyamidoamine dendritic polymer (PAMAM) Antibacterial activity of Escherichia coli and Staphylococcus aureus [105] Ginger, cumin, black pepper EOs Electrospun nanofiber films Antifungal activity against Aspergillus spp. and Penicillium spp. [106] Zanthoxylum bungeanum EOs Polyvinyl alcohol/β-Cyclodextrin (PVA/β-CD, 6:1) nanofibers Antifungal package materials to improve the shelf life of fruits [64,107] Ocimum gratissimum L. and Ocimum basilicum L. EOs Poly(lactic acid) (PLA) nanofibers Antifungal activity against Aspergillus carbonarius and Aspergillus niger [108] Citrus reticulata EO Nanocapsules Antioxidant activity, antifungal activity [77] Thyme oil and betel leaf oil Polyvinyl alcohol nanofibers Disease control on anthracnose disease [109] Lemon grass oil Nanofibers Antifungal activity [110] Zataria multiflora Boiss (ZMEO) Electrospun gliadin nanofibers Antibacterial activity against Escherichia coli and Pseudomonas aeruginosa, Bacillus cereus and Staphylococcus aureus [111] Callistemon viminalis (EOs). IC (EOC/β-CD) Antibacterial, antifungal, and antioxidant activity [112] Inclusion complex based
on β-cyclodextrin (β-CD)
and LCEOAntimicrobial activities against pathogens Penicillium italicum, Penicillium digitatum, and Geotrichum citri-aurantii [113] Different nano-encapsulated EOs show different extents of antifungal activity. Ch-NP encapsulation is famous for its antifungal effects. Hasheminejad et al.[75] reported a growth inhibition trend with free EOs showing no inhibition, whereas CEO-laden Ch-NPs showed 100% inhibition at half-low concentration. It can be concluded that the nanoparticles encapsulating EOs can possess their own antifungal activity. Lipid-based nanosystems have been studied extensively and reported antifungal properties five times better than free EOs, among all the lipid-based Nonencapsulated system nanoliposomes showed more effective results in suppressing the growth of fungi than NEs EOs containing the same EOs[76]. The antifungal activity of various nonencapsulated EOs is shown in Table 1.
Antioxidant activity
-
EOs possess antioxidant activity by scavenging free radicals to maintain food quality, specifically fats and oils, by preventing oxidative damage to food components. Encapsulating EOs in nanocarriers can enhance and preserve this activity by protecting active compounds from degradation, solubility, and controlled release ability. The antioxidant activity of nonencapsulated EOs can be used to prolong the shelf life of products and maintain nutritional quality and flavor by avoiding oxidative spoilage over time and neutralizing free radicals. Recent studies have shown that nano-encapsulated EOs possess high antioxidant activity as compared to unstable volatile EOs. Ali Mahdi et al.[77] encapsulated Citrus reticulata and clove EOs into nanocapsules and concluded that the antioxidant capacity of both EOs was increased by 4.43 and 3.52 times, respectively. Chaudhari et al.[78] proposed nanoemulsions of Origanum majorana EOs (OmEO) as a novel food preservative by enhancing its antioxidant capacity (IC50 = 14.94 and 5.53 μL/mL for DPPH and ABTS, respectively). Dehghan et al.[64] enhanced the shelf life of soya bean oil by preparing emulsions of orange peel essential oil (OPEO) and compared its application results as raw EOs to conclude that nano-encapsulated EOs were able to control peroxide and thiobarbituric acid values in soya bean oil better than non-encapsulated EOs. Hadian et al.[79] prepared nanoparticles (β-CD) with Rosa damascena essential oil REO to enhance antioxidant activity in beverages to improve food applications such as s packaging and storage quality. Nano chitosan coatings were prepared by encapsulating cumin EOs to preserve the quality of the sardine fillet[80].
Insecticidal activity
-
Plant pests are a major problem that can also reduce the yield significantly, the use of chemical insecticides can cause diseases, and be toxic to human beings. EOs are environmentally friendly and toxic to only pests and insects. To enhance their stability towards heat, moisture, and oxygen to make them more soluble in water for consumption, nanoencapsulation can be used in the food sector. Ibrahim et al.[81] encapsulated citronella essential oil against cotton leafworm Spodoptera littoral to enhance the yield by avoiding pests. Ahsaei et al.[82] encapsulated Rosmarinus officinalis and Zataria multiflora EOs to control flour beetles for a long period of time under sustained conditions. Suresh et al.[83] encapsulated sea fennel EOs to treat crop pest Spodoptera litura and dengue vector Aedes aegypti into nanoemulsions to target human diseases and reduce agriculture damage from larva production. Chitosan nanoparticles containing peppermint EOs control grain pests for longer storage periods as grains are seasonal, and their storage is the most important factor in many countries. Management of storage grain flour and other stable foods is increasing, and nano pesticides are being applied to reduce the loss. Nano and microemulsions, inorganic nanomaterial (metal), and polymer-based nano-formulation, which, polymer nanoparticle-based encapsulation of EOs, are the most common applications in the food industry[83].
Flavor and aroma preservation
-
Aromatic compounds have been used for centuries as food additives, but their use has been limited due to the various limitations discussed above in detail. Nanoencapsulation has opened new doors for their consumption in the food industry by protecting flavor and aromas from external factors with controlled release from material to packaged food. Fresh aromas from plants can be used to mask the unwanted smell of different foods and keep them fresh by avoiding spoilage, Hasani et al.[84] encapsulated lemon oil to preserve meat, the soothing aroma of lemon oil can give the product a more attractive smell by keeping it fresh for a longer time. Jayari et al.[85] replaced synthetic additives by applying thyme oil nanoencapsulated into emulsions and nanoparticles for consumption in food systems such as beverages and fat-rich food (gravies, creams, dressing, and mayonnaise) as both food preservatives with flavor. Nanoencapsulation has simplified the use of plant aromas in food science for various purposes, such as providing antimicrobial and antioxidant properties and a refreshing flavor and aroma.
-
The nanoencapsulation of EOs is one of the most promising solutions for avoiding chemical additives and preservatives in the food industry. The introduction of nanoencapsulation in the food industry is still in its infancy, with great potential yet to be explored. The ongoing research on the nanoencapsulation of EOs has been expedited by the growing shift towards clean-label products. This transition is underpinned by heightened consumer awareness and regulatory considerations. Encapsulating these natural compounds at the nanoscale has the potential to enhance their stability, bioavailability, and controlled release. These compounds are widely acknowledged for their antimicrobial and antioxidant properties. However, to widely apply nanoencapsulation of EOs in the food industry, a number of significant challenges need to be addressed. Future studies must focus on the thorough toxicological evaluation of nanocarriers and EO consumption to ensure safe consumption by consumers. Another main research area should focus on understanding the compatibility and interaction of nanocarriers on the nutritional and sensory properties of food products in different food matrices. The change in organoleptic properties such as aroma, taste, and texture due to nanoencapsulation should be studied, controlled, and monitored closely to ensure consumer satisfaction. The challenges at hand can be effectively addressed by implementing the latest advancements in encapsulation methods and materials. It is strongly advised to utilize biocompatible, non-toxic, and biodegradable materials with a high encapsulation ability for EOs. Moreover, the meticulous optimization of experimental conditions during encapsulation, which includes crucial factors such as particle size, surface charge area, and encapsulation efficiency, is essential for ensuring the effective application of nanoencapsulation in food systems.
Future research should unequivocally prioritize the impact of nanoencapsulation on real-world conditions such as shelf life, safety, and functionality of food products. Additionally, a comprehensive analysis of the behavior of nanoencapsulated EOs during food processing, packaging, and storage is imperative to gain invaluable insights into their practical applications as natural preservatives. Moreover, delving into the potential synergistic interactions between nanoencapsulated EOs and other natural preservatives or food components holds great promise for the development of multifunctional food additives.
Studies unequivocally demonstrate that nanoemulsions significantly enhance EOs solubility, stability, and bioavailability, leading to a marked improvement in their antioxidant and antimicrobial properties. However, it is important to acknowledge that these studies often rely on simulated food systems with simplified structures that may not fully capture the complexities of real food products. Therefore, delving into applying nanoemulsions of EOs across diverse and intricate food matrices, such as beverages, solid food systems, and emulsified products, promises to yield crucial insights into their practical implementation and commercial viability.
Further research endeavors should be directed toward addressing the practical challenges associated with the implementation of nanoencapsulation in real-world food and beverage systems. In conclusion, utilizing nanoencapsulation for EOs exhibits substantial promise within the food industry. Through collaborative efforts among experts spanning food science, nanotechnology, toxicology, and industry, the complete integration of nanoencapsulation in the food sector represents an opportunity to transform food preservation. This can lead to the development of safer, more sustainable, and higher-quality food products, thereby addressing current challenges and fostering innovation.
Safety assessment of Nanoencapsulated Essential Oils (NEOs)
-
Applying nanoencapsulated EOs in the food industry significantly challenges consumer safety. Regulatory authorities confidently recognize EOs as generally safe (GRAS); however, the process of nanoencapsulation introduces new variables that demand comprehensive assessment. The primary emphasis lies in conducting comprehensive studies on the toxicity, biodegradation, and biocompatibility of carriers utilized in the nanoencapsulation process. This includes a thorough examination of polymer-based, lipid-based, and other nanostructures employed as nano vehicles within the human body. The utilization of nanocarriers demonstrates the potential to enhance the bioavailability and stability of encapsulated EOs. Furthermore, they can influence pharmacokinetics and bio-distribution, resulting in various interactions within the body. Notably, these interactions may include the ability to penetrate critical biological barriers that larger particles cannot breach, thereby leading to long-term effects on consumers' health. Moreover, comprehensive long-term studies to explore the effects of these nanocarriers have not been undertaken by scientists. Therefore, a detailed toxicological investigation is warranted to assess the safety of both the nanocarriers and the encapsulated EOs. This study should encompass crucial factors such as dosages, exposure duration, and the potential accumulation of nanosized carriers in tissues, owing to their minute scale.
Regulations related to the consumption or application of nano-encapsulated EOs. Despite some nations enacting specific regulations, there is currently no universal consensus on using nanomaterials in food, potentially resulting in divergent safety requirements. Therefore, implementing a comprehensive safety assessment approach is imperative to effectively monitor the behavior of NEOs in various food matrices and inside the human body. This process should involve both in vitro and in vivo research and advanced analytical tools.
-
EOs from herbs have various biological activities, but the volatility and instability limit their practical applications at appropriate levels. Nanoencapsulation technology can effectively encapsulate EOs to avoid early release and achieve long-term effects. However, some issues, such as the quantity, extract cost, as well as specific application scenarios, need to be studied in depth. In the future, the development of novel nanoencapsulation technologies and further study based on the biological activities of EOs will be expected to facilitate the deep integration of basic research with practical applications.
-
The authors confirm their contribution to the paper as follows: writing - original draft, editing, figures: Sheikh AR; writing - review & editing: Wu-Chen RA, Javed M, Matloob A; resources: Matloob A; figures and language editing: Mahmood MH, Sheikh AR. All authors reviewed the results and approved the final version of the manuscript.
-
The data supporting the findings of this study are available upon reasonable request from the corresponding author. All relevant data generated or analyzed during this study have been included in this published article.
The authors would like to acknowledge the support from the Key Research and Development Program of Zhejiang Province, China (2023C02042).
-
The authors declare that they have no conflict of interest.
- Copyright: © 2024 by the author(s). Published by Maximum Academic Press on behalf of China Agricultural University, Zhejiang University and Shenyang Agricultural University. This article is an open access article distributed under Creative Commons Attribution License (CC BY 4.0), visit https://creativecommons.org/licenses/by/4.0/.
-
About this article
Cite this article
Rehman Sheikh A, Wu-Chen RA, Matloob A, Mahmood MH, Javed M. 2024. Nanoencapsulation of volatile plant essential oils: a paradigm shift in food industry practices. Food Innovation and Advances 3(3): 305−319 doi: 10.48130/fia-0024-0028
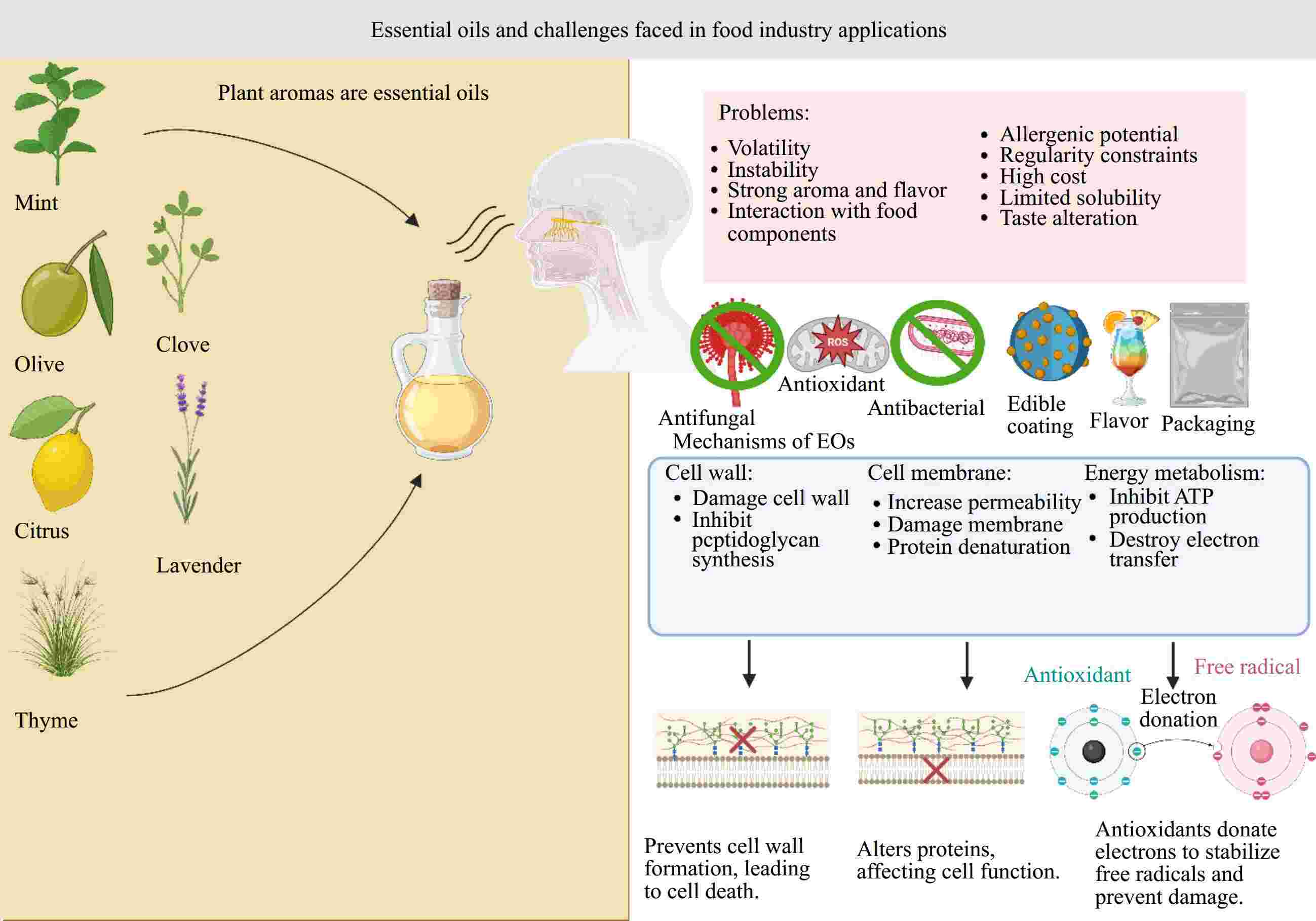