-
Phenolic compounds, which are secondary metabolites in plants, have received increased attention in recent years due to their importance in human nutrition. These bioactives are abundant in a variety of dietary sources, including fruits, vegetables, cereals, dry legumes, and beverages including tea, coffee, and red wine[1]. Their potent antioxidant properties, which include neutralizing free radicals and inhibiting oxidative stress, have been related to the prevention of chronic diseases, including cancer, cardiovascular diseases, gastrointestinal disorders, and neurodegenerative conditions[2]. This renewed interest in phenolic compounds originates from their broad potential to promote health and prevent disease, making them critical components in food science and health research[3].
Red cabbage (Brassica oleracea L. var. capitata f. rubra), a member of the Brassicaceae family, is well-known for its high content of bioactive compounds such as anthocyanins, glucosinolates, carotenoids, and tocopherols[4,5]. It is cultivated globally, and the valorization of this vegetable, along with its by-products or waste, holds substantial importance. Among the vegetables rich in anthocyanins, red cabbage has emerged as one of the most used sources for food production due to its wide pH stability, making it an excellent natural food colorant[6,7]. The anthocyanins in red cabbage have been explored for their antioxidant potential and health-promoting effects, including their role in reducing oxidative stress and mitigating chronic disease risks. This presents a valuable opportunity for the food industry to utilize its phenolic and anthocyanin content as natural additives in various food products[8].
The extraction process is the most crucial step for obtaining polyphenolic extracts with the least possible loss in physicochemical properties[3]. Simple solvent extraction is the most used extraction method for recovering polyphenols, but it is becoming less prevalent, due to its time-demanding nature and large volume of solvents required. Some emergent technologies such as ultrasound, pulsed electric field, high-pressure, microwave, and enzymatic procedures can be alternatives for improving the quality of phenolic extracts while reducing solvent requirements[9]. For the extraction of red cabbage anthocyanins, several methods have been described in the literature. For example, ultrasonic-assisted extraction[10] and pulsed electric field treatment[11] have been investigated for their efficiency in extracting anthocyanins from red cabbage. High-pressure CO2 extraction has also been studied, showing improvements in extraction efficiency compared to conventional methods[12]. Additionally, pressurized solvent extraction has been shown to be an efficient method, yielding rapid results with high anthocyanin content[6,13]. In previous research, it has been demonstrated that enzyme-enhanced extraction can further improve anthocyanin yields from red cabbage[14].
Microwave-assisted extraction (MAE) is an efficient and modern technique that uses microwave energy to induce molecular movements, reducing process times, solvent usage, and energy demand, while increasing extraction yields[15,16]. MAE’s rapid heating, enhanced mass transfer, and minimal solvent consumption make it both environmentally friendly and highly effective for bioactive compound extraction. MAE is particularly advantageous for complex herbal matrices, preserving thermolabile compounds and minimizing environmental impact as Bagade & Patil[17] have highlighted. Despite its proven benefits in various matrices, such as eggplant peel[18], black carrot pomace[19], and Ray Ruby grapefruit leaves[20], the optimization of MAE for red cabbage remains largely unexplored.
As a previous part of this work, MAE conditions for extracting anthocyanins from red cabbage were optimized using response surface methodology (RSM), and the main anthocyanins were characterized using HPLC-MS in the study of Yiğit et al.[8]. This present study has extended this work by focusing on optimizing MAE conditions for total phenolic content and antioxidant capacity, aiming to maximize both. Additionally, structural changes in the extracts obtained using MAE and conventional extraction methods were examined through scanning electron microscopy (SEM). The novelty of this research lies in its specific focus on optimizing MAE for red cabbage phenolics, which is an area largely unexplored in the current literature. While previous studies have demonstrated MAE’s effectiveness for other plant matrices, this research fills a critical gap by applying the technology to red cabbage, contributing new insights for the food and nutraceutical industries. By optimizing this eco-friendly method, the study offers sustainable solutions for a possible future large-scale production of phenolic-rich extracts, with potential applications as natural antioxidants and colorants.
-
Red cabbages (Brassica oleracea L. var. capitata f. rubra) were purchased from a local market in Ankara, Turkey. The leaves of the red cabbage were frozen at −80 °C for 24 h, and they were freeze-dried for 36−48 h by using a lyophilizator (Christ2B, Osterode am Harz, Germany). Dried samples were ground by a coffee grinder (Sinbo, Istanbul, Turkey) and passed through a sieve having a 450 μm mesh opening. They were stored at 4 °C until further analysis and extraction.
Methanol, ethanol, acetic acid, NaOH, gallic acid, Folin-Ciocalteu and copper (II) chloride were purchased from the Merck Chemical Reagents Company (Darmstadt, Germany). Ammonium acetate, sodium carbonate, KCl, sodium acetate, hydrochloric acid, DPPH (2,2-diphenyl-1-picrylhydrazyl), Trolox, and neocuproine were purchased from Sigma-Aldrich (Darmstadt, Germany).
Conventional extraction (CE) of phenolic compounds from red cabbages
-
Maceration was performed as the conventional method by using a shaking water bath (Memmert WNB, Schwabach, Germany). Water and ethanol-water (50:50, v:v) were used as solvents. Five grams of lyophilized red cabbage were macerated at two different temperatures (40 and 70 °C) and for two different time periods (4 and 6 h). Sample/solvent ratio was 1:40. The pH of the samples was adjusted to the range of 3.0−3.3 before the maceration[21]. After maceration, the solution was filtered through Whatman No.1 paper under vacuum. The supernatant was also filtered by 0.45 μm syringe filter and stored for the analysis.
Microwave-assisted extraction (MAE) of phenolic compounds from red cabbages
-
MAE was performed by using a domestic-type microwave oven (MD 20MB, Vestel, İstanbul, Turkey) and a condenser was connected to the upper part of this oven for extraction and the sample and solvent was placed in a flask assembled in the oven (Fig. 1). Five grams of lyophilized and ground red cabbage powder was suspended in extraction solvent in three different solid/solvent ratio (1:20, 1:30, and 1:40, w:w) in the flask and mixed with a stirrer for a good penetration. The solid:solvent ratios were determined by preliminary extraction trials. Two types of solvents were used (water and ethanol-water, 50:50, v:v). The pH of of the solvents were adjusted to 3.0−3.3. Three extractions times, which were 5, 10, and 15 min were applied at three different microwave irradiation powers (200, 400, and 600 watt). These power levels were selected based on both the equipment's capabilities and preliminary trials, which indicated optimal phenolic extraction across this range. Although a household microwave oven was used for the experiments, the selected power levels are consistent with those commonly used in industrial extraction processes, as both settings operate at the same frequencies and power ranges. According to the IMPI (International Microwave Power Institute) 2 L test, the real powers absorbed by the sample were 298.3, 366.4, and 467.6 watt for low, medium, and high levels)[22]. After each extraction step, the flask containing solid, and solvent was taken out of the oven and cooled to room temperature under the tap water. Then, the solution was filtered through Whatman No.1 paper under a vacuum. The supernatant was also filtered by 0.45 μm syringe filter and stored for analysis.
Total phenolic content (TPC)
-
Total phenolic contents of liquid extracts obtained from CE and MAE methods were determined by Folin-Ciocalteu method[23]. The extracts were diluted to a ratio of 1:20 by using water or ethanol-water mixture (50:50, v:v). 2.5 ml Folin-Ciocalteu reagent (0.2 N) was added to 0.5 ml sample. The mixture was vortexed for 5 s and kept in the dark for 5 min. Two ml sodium carbonate solution (75 g/L) was added to the mixture, and it was vortexed. This last mixture was kept in dark for 1 h. Then the absorbance was measured in a spectrophotometer at 760 nm. A calibration curve of gallic acid for different concentrations was prepared and the results were expressed as mg GAE/L (GAE : gallic acid equivalent).
Total antioxidant capacity (TAC)
DPPH assay
-
This analysis was applied according to the method described by Akdeniz et al.[23]. The extracts were diluted to a ratio of 1:20 by using water or ethanol-water mixture (50:50, v:v). 3.9 ml DPPH solution (25 ppm) was added to 100 μl sample. The mixture was vortexed for 5 s and kept in the dark for 1 h. Then the absorbance was measured in a spectrophotometer at 517 nm. A calibration curve of DPPH for different concentrations (5, 10, 15, 20, and 25 ppm) was prepared and the results (TACDPPH) were expressed as mg DPPH/L.
CUPRAC assay
-
This analysis was applied according to Özyürek et al.[24]. Firstly, 0.2 M copper (II) chloride solution, 1 M and pH 7.0 ammonium acetate solution and 7.5 × 10−3 M neocuproin solution were prepared. The extracts were diluted to a ratio of 1:60 by using water or ethanol-water mixture (50:50, v:v). One ml copper (II) chloride solution, 1 ml neocuproin solution, 1 ml ammonium acetate solution and 1.1 ml water were mixed with 10 ml diluted solid sample, and this was vortexed for 5 s. It was kept in the dark for 1 h and then, then the absorbance was measured in a spectrophotometer at 450 nm. A calibration curve of Trolox for different concentrations (50, 100, 150, 200, and 250 ppm) was prepared and the results (TACCUPRAC) were expressed as mmol TE/L (TE : Trolox equivalent).
Scanning electron microscopy (SEM)
-
The microstructure of red cabbage extracts from conventional and microwave-assisted extractions was investigated using a scanning electron microscope (SEM) (Gaia 3, Tescan). The extracts were lyophilized before the analysis to remove the moisture inside. The samples were coated with a thin layer of gold and mounted on stubs with the help of a double-sided adhesive tape before the visualization[25]. The SEM images were taken at 100×, and 2,500× magnifications.
Experimental design and statistical analysis
-
Response surface methodology (RSM) was applied to optimize the MAE of phenolic compounds from red cabbage and Box-Behnken experimental design was employed for the experimental design. Extraction time (X1), solid/solvent ratio (X2), and microwave power (X3) were chosen as independent variables. The range and center point values of three independent variables presented in Table 1 are chosen according to preliminary experiments. The complete experimental design consisted of 17 experimental points containing 12 factorial points and five center points are shown in Supplementary Tables S1 & S2 for both solvent types, which are water and ethanol-water (50:50, v:v). Experimental runs were randomized to minimize the unexpected variability.
Table 1. Coded and actual values of the independent variables.
Independent variables Code levels −1 0 1 Extraction time (X1, min) 5 10 15 Solid : solvent ratio (X2, 1/X) 20 30 40 Microwave power (X3, W) 200 400 600 The mathematical model corresponding to the Box-Behnken design (Eqn 1) was partitioned into linear, quadratic, and interactive components.
Y=b0+∑ki=1biXi+∑ki=1biiX2i+∑k−1i=1∑kj>1bijXiXj (1) where, Y is the predicted response (TPC, TACCUPRAC and TACDPPH), b0 is a constant and bi, bii and bij are the model coefficients. They represent the linear, quadratic and interaction effects of variables. The adequacy of the model was determined by evaluating the lack of fit, coefficient of determination (R2), and the Fischer test value (F-value) obtained from the analysis of variance (ANOVA) that was generated by Design Expert 12.0 statistical software. One-way ANOVA and Tukey analysis were also performed.
-
The maximum total phenolic content (TPC), and total antioxidant capacity values (TACDPPH and TACCUPRAC) obtained from MAE (solvent:water) were 2154.1 mg GAE/L (15 min, 1:20 solid : solvent ratio, 400 watt), 136.1 mg DPPH/L (15 min, 1:20 solid : solvent ratio, 400 watt), and 22.3 mmol TE/L (5 min, 1:20 solid : solvent ratio, 400 watt), respectively (Supplementary Table S1). The minimum values were 651 mg GAE/L (5 min, 1:40 solid : solvent ratio, 400 watt), 32.5 mg DPPH/L (5 min, 1:40 solid : solvent ratio, 400 watt), and 4.36 mmol TE/L (5 min, 1:40 solid : solvent ratio, 400 watt) for the same solvent (Supplementary Table S1). Supplementary Table S2 shows the experimental results for the ethanol-water mixture solvent. According to these results, the maximum values for TPC, TACDPPH, and TACCUPRAC were 1,106.4 mg GAE/L (10 min, 1:20 solid : solvent ratio, 200 watt), 134.4 mg DPPH/L (10 min, 1:20 solid : solvent ratio, 600 watt), and 17.3 mmol TE/L (10 min, 1:20 solid : solvent ratio, 600 watt), respectively. On the other hand, the minimum values for the same solvent were 634.5 mg GAE/L (5 min, 1:40 solid : solvent ratio, 400 watt), 60.8 mg DPPH/L (15 min, 1:40 solid : solvent ratio, 400 watt), and 6.6 mmol TE/L (10 min, 1:40 solid : solvent ratio, 600 watt).
Fitting the model equations
-
RSM was applied for the optimization of MAE process conditions for obtaining the maximum yields for total phenolics (TPC) and total antioxidant capacities (TACDPPH and TACCUPRAC). Two identical Box-Behnken designs, each containing 17 experiments, were performed for two types of solvents, which were water and ethanol-water mixture (50:50, v:v). Extraction time (X1), solid/solvent ratio (X2), and microwave power (X3) were the independent variables chosen for maximizing the yields.
Design matrices of the experimental conditions with their corresponding response values for both types of solvents were subjected to regression analysis, and the significance of each coefficient in the models was calculated (Supplementary Tables S1 & S2). Design Expert 12.0 software recommended the quadratic models with regression for all responses, and the adequacies of the models were confirmed using variance analysis (ANOVA) for TPC, TACDPPH, and TACCUPRAC. Tables 2 & 3 summarize ANOVA analysis for these responses obtained from MAE with water and water-ethanol mixture solvents, respectively.
Table 2. ANOVA for the quadratic equations of Design Expert 12.0.1 for MAE of TPC, TACDPPH, and TACCUPRAC in red cabbage and statistical indicators for each response (solvent : water).
Source Sum of squares df Mean square F-value p-value Model (TPC) 2.34E+06 8 2.92E+05 67.92 0.0001 Significant X1 20274.94 1 20274.94 4.72 0.0819 X2 1.69E+06 1 1.69E+06 392.13 < 0.0001 X3 22967.4 1 22967.4 5.34 0.0688 X1X2 22734.61 1 22734.61 5.29 0.0698 X1X3 12778.04 1 12778.04 2.97 0.1453 X2X3 3159.87 1 3159.87 0.7352 0.4304 X12 26836.11 1 26836.11 6.24 0.0546 X22 2.33E+05 1 2.33E+05 54.26 0.0007 X32 0 0 Residual 21488.64 5 4297.73 Lack of fit 14143.94 1 14143.94 7.7 0.0501 Not significant Pure error 7344.7 4 1836.18 Cor total 2.36E+06 13 Model (TACDPPH) 9307.92 8 1163.49 4871.42 < 0.0001 Significant X1 193.06 1 193.06 808.33 < 0.0001 X2 6939.72 1 6939.72 29055.95 < 0.0001 X3 29.43 1 29.43 123.22 0.0001 X1X2 306.43 1 306.43 1282.97 < 0.0001 X1X3 262.93 1 262.93 1100.85 < 0.0001 X2X3 16.56 1 16.56 69.31 0.0004 X12 14.59 1 14.59 61.1 0.0005 X22 708.01 1 708.01 2964.36 < 0.0001 X32 0 0 Residual 1.19 5 0.2388 Lack of fit 0.5408 1 0.5408 3.31 0.143 Not significant Pure error 0.6534 4 0.1633 Cor total 9309.12 13 Model (TACCUPRAC) 184.87 8 23.11 16.62 0.0033 Significant X1 5.4 1 5.4 3.88 0.106 X2 109.62 1 109.62 78.82 0.0003 X3 1.45 1 1.45 1.04 0.3537 X1X2 55.2 1 55.2 39.7 0.0015 X1X3 1.78 1 1.78 1.28 0.309 X2X3 1.23 1 1.23 0.8834 0.3904 X12 0.7006 1 0.7006 0.5038 0.5096 X22 1.42 1 1.42 1.02 0.3587 X32 0 0 Residual 6.95 5 1.39 Lack of fit 3.91 1 3.91 5.13 0.0863 Not significant Pure error 3.05 4 0.7619 Cor total 191.83 13 Table 3. ANOVA for the quadratic equations of Design Expert 12.0.1 for MAE of TPC, TACDPPH, and TACCUPRAC in red cabbage and statistical indicators for each response (solvent : ethanol-water, 50:50, v:v).
Source Sum of squares df Mean square F-value p-value Model (TPC) 2.80E+05 9 31099.63 7.02 0.0088 Significant X1 19499.18 1 19499.18 4.4 0.0741 X2 1.93E+05 1 1.93E+05 43.66 0.0003 X3 6063.76 1 6063.76 1.37 0.2803 X1X2 2.36 1 2.36 0.0005 0.9822 X1X3 7959.32 1 7959.32 1.8 0.222 X2X3 359.1 1 359.1 0.0811 0.7841 X12 42236.73 1 42236.73 9.53 0.0176 X22 9986.68 1 9986.68 2.25 0.1769 X32 2963.88 1 2963.88 0.669 0.4403 Residual 31011.78 7 4430.25 Lack of fit 25791.29 3 8597.1 6.59 0.0501 Not significant Pure error 5220.5 4 1305.12 Cor total 3.11E+05 16 Model (TACDPPH) 8898.31 9 988.7 21.78 0.0003 Significant X1 0.2048 1 0.2048 0.0045 0.9483 X2 7871.99 1 7871.99 173.4 < 0.0001 X3 7.09 1 7.09 0.1561 0.7045 X1X2 19.98 1 19.98 0.4401 0.5283 X1X3 3.96 1 3.96 0.0872 0.7763 X2X3 3.55 1 3.55 0.0783 0.7877 X12 432.95 1 432.95 9.54 0.0176 X22 533.11 1 533.11 11.74 0.011 X32 75.26 1 75.26 1.66 0.2388 Residual 317.79 7 45.4 Lack of fit 66.7 3 22.23 0.3542 0.7899 Not significant Pure error 251.08 4 62.77 Cor total 9216.09 16 Model (TACCUPRAC) 167.95 9 18.66 25.72 0.0001 Significant X1 0.4512 1 0.4512 0.622 0.4562 X2 137.7 1 137.7 189.81 < 0.0001 X3 2.59 1 2.59 3.57 0.1009 X1X2 1.24 1 1.24 1.71 0.2318 X1X3 0.0462 1 0.0462 0.0637 0.808 X2X3 5.62 1 5.62 7.74 0.0272 X12 3.09 1 3.09 4.26 0.0779 X22 16.41 1 16.41 22.63 0.0021 X32 0.1155 1 0.1155 0.1592 0.7018 Residual 5.08 7 0.7254 Lack of fit 3.32 3 1.11 2.51 0.1973 Not significant Pure error 1.76 4 0.44 Cor Total 173.03 16 The models obtained for total phenols (TPC) in terms of mg GAE/L were significant with p-value = 0.0001, with R2 value of 0.9909 and adjusted R2 value of 0.9763 for water and p-value = 0.0088, with R2 value of 0.9003 and adjusted R2 value of 0.7720 for ethanol-water mixture. Sin et al.[26] reported that an R2 value greater than 0.8 is desirable for a developed regression model. The lack of fit values was not significant (p > 0.05), showing that the models were well-fitted and could be used to predict the TPC of the extracts. By applying multiple regression analysis to the experimental data of TPC for both solvents, the following second-order polynomial equations (Eqns 2 & 3) were found:
TPC(water)=1016.63−649.09X2+341.46X22 (2) TPC(ethanol-water)=858.95−155.50X2−100.16X21 (3) The models obtained for total antioxidant capacity (TACDPPH) in terms of mg DPPH equivalent/L were significant with p-value < 0.0001, with R2 value of 0.999 and adjusted R2 value of 0.999 for water and p-value = 0.0003, with R2 value of 0.966 and adjusted R2 value of 0.921 for ethanol-water mixture. The lack of fit values was not significant (p > 0.05), showing that the models were well-fitted and could be used to predict TACDPPH of the extracts. By applying multiple regression analysis to the experimental data of TACDPPH for both solvents, the following second-order polynomial equations (Eqns 4 & 5) were found:
TACDPPH(water)=66.67+4.91X1−41.65X2−2.71X3+8.75X1X2+8.11X1X3−6.04X2X3+2.56X21+18.82X22 (4) TACDPPH(ethanol-water)=96.28−31.37X2−10.14X21+11.25X22 (5) Total antioxidant capacity (TACCUPRAC) models in terms of mmol TE/L were significant with p-value = 0.0033, with R2 value of 0.9638 and adjusted R2 value of 0.9058 for water and p-value = 0.0001, with R2 value of 0.9707, and adjusted R2 value of 0.9329 for ethanol-water mixture. The lack of fit values was not significant (p > 0.05). By applying multiple regression analysis to the experimental data of TACCUPRAC for both solvents, the following second-order polynomial equations (Eqns 6 & 7) were found:
TACCUPRAC(water)=10.39−5.24X2+3.72X1X2 (6) TACCUPRAC(ethanol−water)=9.06−4.15X2−1.18X2X3+1.97X22 (7) Response surface graphs
-
The response surface graphs for TPC results for the solvent types of water (Fig. 2a & b) and ethanol-water (Fig. 2c & d) were illustrated in Fig. 2. Figures 3 & 4 show the response surfaces for TACDPPH and TACCUPRAC, respectively. In all these figures, the effects of extraction parameters on dependent variables can be seen, compared, and the interaction of different parameters can be discussed.
Figure 2.
Response surfaces for TPC graphs (a) and (b) are for solvent : water; graphs (c) and (d) are for solvent : ethanol-water.
Figure 3.
Response surfaces for TACDPPH, graphs (a) and (b) are for solvent:water; graphs (c) and (d) are for solvent:ethanol-water.
Figure 4.
Response surfaces for TACCUPRAC graphs (a) and (b) are for solvent : water; graphs (c) and (d) are for solvent : ethanol-water.
These figures generally corroborate previous research and the models of this work given in the previous section emphasizing the significance of solid : solvent ratio and its interactions with other variables for optimizing the extraction of bioactive compounds. Studies have shown that fine-tuning the solid : solvent ratio can significantly enhance phenolic compound yield[27]. The interaction effects underscore the complexity of the extraction process, where multiple factors interact to influence phenolic compound recovery efficiency[28].
Conventional extraction of red cabbage phenolics
-
The conventional extraction (CE) of phenolic compounds from red cabbage using water and an ethanol-water mixture at varying temperatures (40 and 70 °C) and extraction times (4 and 6 h) showed distinct patterns. At 40 °C with water, TPC increased with longer extraction times, while at 70 °C, TPC decreased after 6 h, suggesting thermal degradation[16,27]. Ethanol-water mixtures provided higher TPC values, but a decrease was observed at 70 °C for longer extraction times. Similarly, antioxidant capacity (TACCUPRAC and TACDPPH) values were higher at 40 °C for 4 h and decreased at higher temperatures and longer times, indicating potential degradation[14,29].
In comparison, microwave-assisted extraction (MAE) demonstrated superior efficiency over CE. MAE significantly reduced extraction times while yielding higher TPC and antioxidant capacity values. Using ethanol-water, MAE achieved 1,062.6 mg GAE/L TPC in just over 9 min, whereas CE required 4−6 h to reach its maximum of 1,677.3 mg GAE/L. With water, MAE yielded 2,146 mg GAE/L TPC in 5 min, surpassing CE. The same trend was observed in antioxidant capacities, where MAE achieved higher TACCUPRAC and TACDPPH values in shorter times. The rapid and uniform heating provided by MAE contributed to this efficiency[28,30].
Microstructure evaluation
-
SEM images in Fig. 5a & b show red cabbage extract samples obtained from optimal conditions at CE. Rough and uneven surface morphology with a wide range of particle sizes can be seen in Fig. 5a. This heterogeneity in surface texture may indicate the insufficient breakdown of cell walls and incomplete release of intracellular compounds. At higher magnification (2,500×), Fig. 5b displays a more granular surface, indicating that the CE approach may leave residual cellular structures and bound phenolics. CE usually involves prolonged heating and solvent penetration, which can lead to incomplete extraction and degradation of sensitive bioactives.
Figure 5.
SEM images of red cabbage extracts. (a) Red cabbage extract from CE (100×), (b) red cabbage extract from CE (2,500×), (c) red cabbage extract from MAE (100×), (d) red cabbage extract from MAE (2,500×)
In contrast, Fig. 5c & d depict red cabbage extracts obtained through optimal conditions at MAE. Figure 5c demonstrates a more uniform particle distribution with smaller and more evenly broken particles than CE. These results suggest that MAE is more efficient at cell wall destruction and releasing phenolic compounds. MAE improves mass transfer rate and extraction efficiency by rapid heating and high energy input. Figure 5d further illustrates the effect of MAE at a magnification of 2,500×, where the surface seems more fibrillar. MAE can cause significant structural changes at the cellular level, leading to more efficient extraction. The dielectric heating effect during MAE causes the fibrillar structure of the extract surface because of the breakdown of the plant cell matrix and the release of bound compounds. The differences in microstructure between CE and MAE extracts, as shown in Fig. 5, indicate the superior efficiency of MAE in breaking down cell walls and enhancing extraction.
-
The extraction of phenolic compounds from plant material is influenced by various factors, including the nature of the plant material, type and concentration of solvent, sample:solvent ratio, particle size, extraction time, and temperature[8]. The interaction of the solvent with microwaves can also significantly impact the extraction process. Ethanol, methanol, and acetone are commonly used as organic solvents due to their high solubility and polarity characteristics[31]. In this study, acidified water (pH 3.0−3.3) and an ethanol-water mixture (50:50, v:v) were used. The solid : solvent ratio was included as an independent variable due to its impact on extraction yield (Table 1). Additionally, microwave irradiation power (watt) and extraction time (min) were considered crucial variables because they influence the temperature inside the extraction vessel.
Model evaluation and implications
-
ANOVA results (Table 2) indicated that the solid:solvent ratio (X2) and its quadratic term (X22) were the most significant factors affecting TPC during MAE when water was used as the solvent. An increase in the solid:solvent ratio had a negative linear impact on TPC, while the quadratic effect was positive. Neither extraction time (X1) nor microwave power (X3) significantly influenced TPC within the studied ranges (5−15 min, 200−600 watt). Similar trends have been reported in other studies, where the solid : solvent ratio was a critical factor for phenolic extraction, while microwave power had a limited effect[27]. Therefore, the solvent type and ratio were two significant effects for phenolic compound extraction[29]. For the ethanol-water mixture (Table 3), the solid : solvent ratio remained a significant factor, along with the quadratic term of extraction time (X12). This indicates that the solid : solvent ratio continues to dominate the process, whereas the role of the quadratic effect of extraction time becomes more influential.
Table 4. TPC, TACCUPRAC and TACDPPH yields of conventional extraction.
Temperature (°C) Time (h) Solvent* TPC (mg GAE/L) TACCUPRAC (mmol TE/L) TACDPPH (mg DPPH/L) 40 4 w 1018.5 ± 1.28D 8.9 ± 0.03B 33.1 ± 0.75B 6 1165.9 ± 1.29C 11.2 ± 0.02A 54.52 ± 0.32A 70 4 1324.4 ± 2.22A 11.6 ± 0.16A 48.0 ± 0.08A 6 1192.6 ± 1.28B 11.6 ± 0.31A 50.2 ± 1.78A 40 4 e-w 1677.3 ± 1.15a 16.77 ± 0.14a 66.3 ± 0.81a 6 1515.3 ± 1.15b 14.2 ± 0.04b 58.5 ± 0.49b 70 4 1268.0 ± 2.00c 10.0 ± 0.03d 36.1 ± 1.48d 6 1202.7 ± 1.15d 10.7 ± 0.03c 44.5 ± 0.65c The results are given as the mean of three replicates with the standard deviations. Different letters represent the significant differences (p ≤ 0.05) between the results for each column. Upper case letters represent the solvent-water and lower case letters represent the solvent-ethanol-water. * w: water, e-w: ethanol-water. Regarding antioxidant capacity (TACDPPH), all independent variables (X1, X2, X3) significantly impacted the values when water was used as the solvent (Eqn 4). Extraction time (X1) had a positive effect on TACDPPH, whereas solid : solvent ratio (X2) and microwave power (X3) had negative effects. The quadratic terms for both extraction time and solid : solvent ratio were significant, suggesting a complex relationship between these variables and antioxidant capacity. Similar findings have been reported by Yiğit et al.[8] in a study on anthocyanin content. For the ethanol-water solvent, the TACDPPH model (Eqn 5) showed that the solid : solvent ratio was the only significant linear variable, while quadratic terms for extraction time (X1²) and solid : solvent ratio (X2²) were also significant. These results align with those from the TPC models. Studies such as those by Rodsamran & Sothornvit[32] similarly noted that microwave power does not significantly affect antioxidant capacity during MAE.
ANOVA results (Table 2) for TACCUPRAC indicated that the solid:solvent ratio (X2) and its interaction with extraction time (X1X2) were significant. Increasing the solid : solvent ratio led to a decrease in TACCUPRAC, although this effect was mitigated when extraction time and solid : solvent ratio were optimized together. Similar trends were observed with the ethanol-water mixture, where the interaction between solid/solvent ratio and microwave power (X2X3), as well as the quadratic effect of solid : solvent ratio (X2²), were significant. The quadratic relationship highlights that increasing the solid : solvent ratio beyond a certain point can positively influence TACCUPRAC.
In future microwave-assisted extraction studies, the microwave power that provides the best results for phenolic extraction may be kept constant, while focusing on optimizing the solid : solvent ratio, solvent type, and extraction time.
Optimization of extraction parameters and response surfaces
-
Optimal conditions for MAE were determined using the desirability function, with values of 0.838 for ethanol-water, and 0.924 for water. X1 = 9.2 min, X2 = 1:20, X3 = 501.8 watt for ethanol-water solvent, and X1 = 5 min, X2 = 1:20, X3 = 384.1 watt for water-only solvent were found as optimum conditions for MAE method. These results showed that lower extraction time and microwave power were required when water is used than when ethanol-water was used the solvent to obtain the optimum phenolic content and antioxidant capacity values. Therefore, this indicated that there were more water-soluble phenolic compounds in the samples. Moreover, higher extraction time or microwave power levels may also cause degradation of possible heat-labile phenolics in the extracts.
The response surface plots for TPC under different conditions of water and ethanol-water solvents are illustrated in Fig. 2. Increasing the solid : solvent ratio significantly improves TPC, particularly at moderate extraction times. Moderate microwave power combined with an optimal solid : solvent ratio enhances TPC, as seen in Fig. 2b[33]. For the ethanol-water mixture, a lower solid : solvent ratio paired with moderate microwave power yields the highest TPC values[27]. These results align with findings indicating that extraction parameters, such as solvent polarity and extraction time, strongly influence phenolic content[6].
Figure 3 shows the response surfaces for TACDPPH. In the water solvent system, a balanced solid : solvent ratio and longer extraction times optimize antioxidant capacity. In contrast, moderate microwave power combined with optimal solid : solvent ratios yields the best results with the ethanol-water mixture. These trends suggest that antioxidant compounds are sensitive to extraction conditions, with higher power settings potentially leading to thermal degradation[30,34]. Studies have similarly noted the importance of controlling microwave power to preserve antioxidant properties[28].
For total antioxidant capacity measured by the CUPRAC assay (TACCUPRAC), Fig. 4 demonstrates that balanced extraction time and solid:solvent ratio maximize the yields in both solvent systems. Lower microwave power and optimal solid:solvent ratios consistently result in higher antioxidant capacity for ethanol-water extracts. These findings underscore the non-linear effects of variables like extraction time and microwave power, which must be carefully controlled to optimize both phenolic and antioxidant recovery[8,11].
Comparison between CE and MAE methods
-
The comparison of CE and MAE techniques revealed that MAE demonstrated superior efficiency in extracting phenolic compounds and antioxidants from red cabbage (Table 4). MAE not only reduced extraction times significantly but also enhanced the yield of phenolic compounds and antioxidants compared to CE. The use of an ethanol-water mixture generally provided better extraction yields than water alone in both methods, with the benefits being more pronounced in MAE[11]. One of the major advantages of MAE is its ability to rapidly heat the solvent and sample, which allows for shorter extraction times. This minimizes the exposure of sensitive phenolic compounds to high temperatures, reducing the risk of thermal degradation[15]. In contrast, CE methods, which typically involve longer extraction times at higher temperatures, may lead to a higher rate of phenolic compound degradation. Reducing microwave power and extraction time in MAE can further mitigate potential damage to sensitive phenolic compounds, providing a distinct advantage over CE in preserving compound integrity[34]. These findings align with previous studies that reported ethanol as an effective solvent for polyphenol extraction due to its polarity and ability to interact with microwaves[28]. Additionally, studies have shown that MAE often achieves better extraction efficiency and shorter processing times compared to ultrasound-assisted extraction (UAE)[32].
Microstructure of CE and MAE extracts
-
The SEM images revealed significant differences in the microstructure of the extracts obtained through CE and MAE. CE extracts exhibited rough and uneven surface morphology with a wide range of particle sizes, suggesting insufficient breakdown of cell walls and incomplete release of intracellular compounds. In contrast, MAE extracts demonstrated more uniform particle distribution with smaller and more evenly broken particles, indicating more efficient cell wall destruction and release of phenolic compounds.
The fibrillar surface structure observed in MAE extracts, as compared to CE, can be attributed to the dielectric heating effect of microwaves, which causes significant structural changes at the cellular level. These findings suggest that MAE is a more effective method for obtaining high yields of phenolic compounds and antioxidants from red cabbage, as it enhances mass transfer rates and extraction efficiency through rapid heating and high energy input.
-
The effectiveness of microwave-assisted extraction (MAE) compared to conventional methods (CE) was demonstrated by this study for obtaining phenolic compounds and antioxidants from red cabbage. Response surface methodology was applied in the optimization of the key extraction parameters, including extraction time, solid/solvent ratio, and microwave power, for both water and ethanol-water solvents. Box-Behnken design was used in experimental design. MAE reduced the required time and enhanced the yield of total phenolic content and antioxidant capacities by improving extraction efficiency. This method proved to be superior to CE, which included longer extraction times and yielded lower concentrations of desired bioactive compounds. Scanning electron microscopy (SEM) images confirmed the structural differences between the two methods, and they showed that MAE-treated samples had more uniform particle distribution and higher porosity. These observations suggest that MAE can break down the cell wall in plants and release intracellular compounds more effectively compared to CE. Overall, the results of this study strongly support the utilization of MAE as an advanced method for the extraction of phenolic compounds and antioxidants from red cabbage. This technology not only reduces processing time but also preserves bioactive compounds, making it suitable for the food industry. Further studies could be recommended for the application of MAE in other plant materials and exploration of its scalability for industrial use. Additionally, investigation of the stability and bioavailability of the extract samples could provide valuable insights into their health benefits and potential applications in food products.
This research was supported by Hacettepe University-Scientific Research Projects Coordination (BAP) Unit (FBA-2017-12587).
-
The authors confirm contribution to the paper as follows: study conception and design: Turabi Yolaçaner E; data collection: Yiğit Ü; analysis and interpretation of results: Turabi Yolaçaner E, Yiğit Ü; draft manuscript. preparation: Turabi Yolaçaner E, Yiğit Ü. Both authors reviewed the results and approved the final version of the manuscript.
-
The datasets generated during and/or analyzed during the current study are available from the corresponding author on reasonable request.
-
The authors declare that they have no conflict of interest.
- Supplementary Table S1 Experimental design by Box-Behnken and the results* of the experiments (Solvent: water).
- Supplementary Table S2 Experimental design by Box-Behnken and the results* of the experiments (Solvent: ethanol-water, 50:50, v:v).
- Copyright: © 2024 by the author(s). Published by Maximum Academic Press on behalf of Nanjing Agricultural University. This article is an open access article distributed under Creative Commons Attribution License (CC BY 4.0), visit https://creativecommons.org/licenses/by/4.0/.
-
About this article
Cite this article
Yiğit Ü, Turabi Yolaçaner E. 2024. Enhanced extraction of phenolic compounds from red cabbage utilizing microwave-assisted method: a Box-Behnken approach for optimization. Food Materials Research 4: e030 doi: 10.48130/fmr-0024-0024
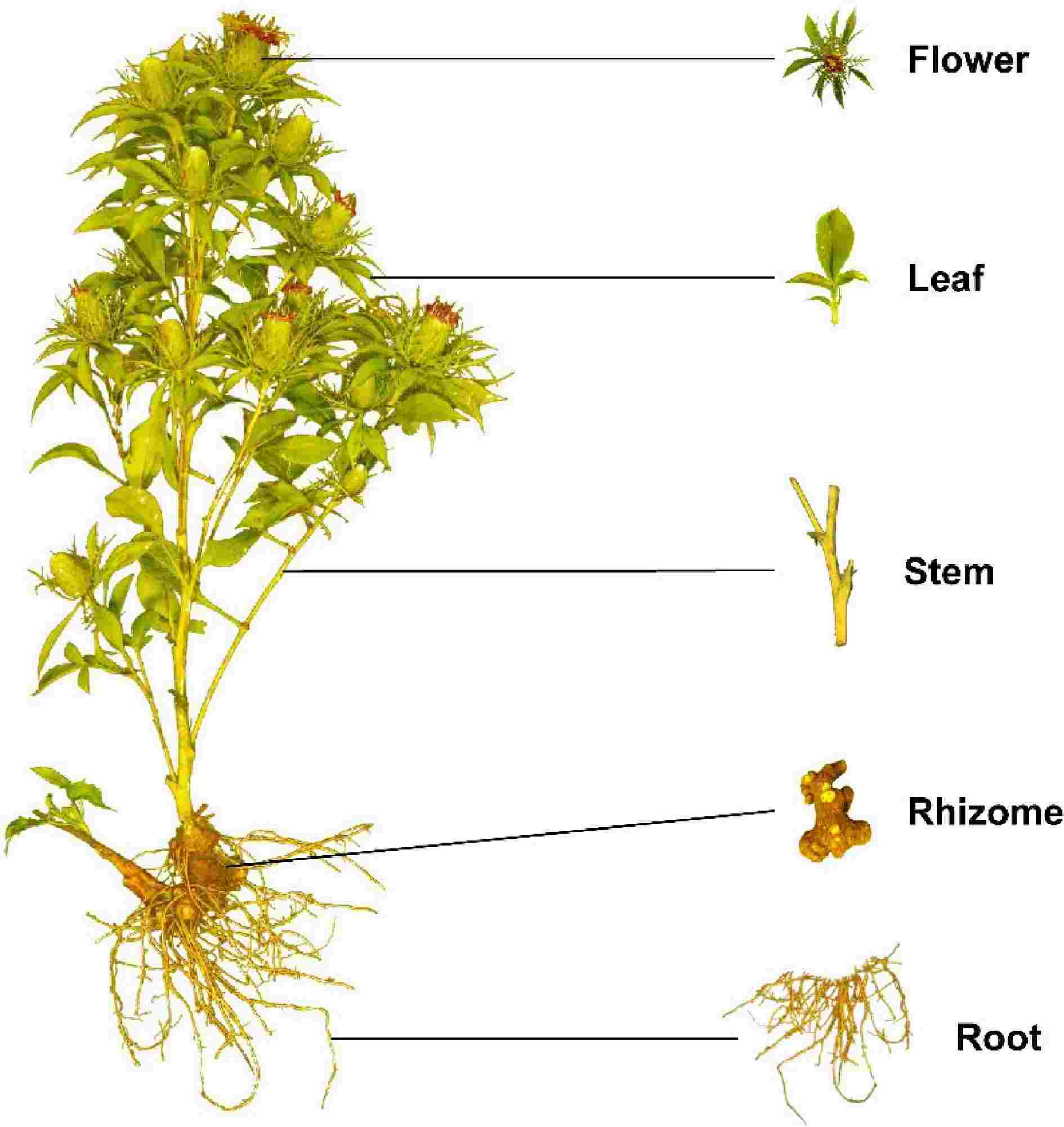